Smart Solids: Zeb Rocklin Awarded NSF CAREER for Flexible Metamaterials Research
Dec 12, 2023 —

Imagine materials that respond to their environment: winter jackets that become thicker as temperatures drop, shoes that return energy with each stride, and robots that adapt to better accomplish their task as they aid in space exploration. All of these ideas could be made into a reality through mechanical metamaterials, a group of flexible solids that blur the traditional definition of what a solid is.
Understanding these metamaterials is key to “programming” them correctly, maximizing their utility. “One of the paradigms of this research is that the material is the machine,” Zeb Rocklin, an assistant professor in the School of Physics, explains. “We're creating a material that performs the mechanical tasks that we want it to, and the processes, forces and displacements in the ways we want it to.”
A new $630,000 NSF CAREER grant will help Rocklin continue that research.
The National Science Foundation Faculty Early Career Development Award is a five-year grant designed to help promising researchers establish a foundation for a lifetime of leadership in their field. Known as CAREER awards, the grants are NSF’s most prestigious funding for untenured assistant professors.
The award, for “Geometric and topological mechanics of flexible structures,” will help Rocklin continue developing a new, unified theory for mechanical metamaterials — a group of structures that can flex and move, while having traditional solid components that make it easier to model. The theory could then be applied by other scientists and engineers to create responsive objects with smart fabrics that could respond to changes in environment — like novel knee replacements, responsive airplane wings, and better robots.
Materials as machines
“A solid is defined by the fact that it has a shape, and if I try to change the shape it might generate patterns of stress, or if I hit it, you might hear noise, because it's vibrating,” says Rocklin. “While we often think about things in terms of solids, liquids, and gasses, a lot of the things that are very important to us are not what we think of as a conventional solid.”
Flexible solids, like clothing, robots, and even our own bodies permeate our world, and are often some of the most useful materials we encounter. “This creates this huge challenge,” Rocklin says, “because flexible solids can't always be understood using current techniques of physics. We can write down the equations, but the equations are often too hard for anyone to solve.” For example, imagine trying to predict or replicate the infinite ways a piece of paper can crumple. As a result, flexible solids are often expensive and time consuming to model.
That’s where Rocklin’s new theory comes in.
Mechanical metamaterials
By combining well-known solids with flexible properties, Rocklin hopes to create a mathematically simple theory. “There are philosophical differences and limitations here,” he says, “but as a physicist, I’m looking for universal principles that can apply to a variety of things. Our technique is meant to complement the existing simulations, and it's meant to provide us more insight into these systems so that we can understand how to control them better.”
By building a theory around materials made of repeating solids connected by flexible hinges, Rocklin hopes to make a computationally inexpensive technique to predict and control the deformation of flexible structures. One example of this type of structure consists of solid square pieces connected by their corners in a checkerboard pattern. The pieces pivot against each other at these hinged corners, allowing the structure to easily expand and contract. “These materials find a sweet spot in between simple solids that were well-characterized in the nineteenth century and the flexible objects that are just too complicated for us to fully describe,” Rocklin adds.
While the material can only deform via one method, (by flexing at the hinges) this does not mean that there is only one way the material deforms. Rather, through this one method of deformation, there are an infinite number of modes or computations that the fabric can assume, illustrating Rocklin's key insight – that a single flexible mode inevitably gives rise to a whole host of complex deformations.
“There's very simple universal math to describe how this type of material operates,” Rocklin adds. “And, when people actually make this material, it turns out that it actually looks like this, and it actually deforms in this way.”
Broad applications
As a theoretical physicist, Rocklin is focused on developing a unified theory that can be applied by experts across many fields. For example, collapsable biomedical devices like stents, which should be small when inserted, but need to expand when inside the body. Inspired by the ever-adapting wings of birds, adaptable airplane wings are also an intriguing frontier.
Rather than minute adjustments via circuitry, airplane wings could be built from these flexible solids, which could be designed to automatically adapt when given a signal from the wind. Building an antenna from materials that respond to certain electromagnetic frequencies, to optimize signal reception, is another of many possible applications for the work.
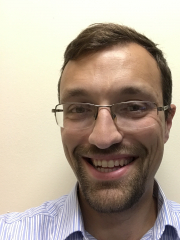
Written by Selena Langner
Contact: Jess Hunt- Ralston