Nature-inspired Strong, Lightweight Material for Planes, Buildings, and Bone Implants
Apr 27, 2022 — Atlanta, GA

The new materials could have applications for reconstructive surgery and other fields.
Material coming from a Princeton research lab is full of holes — but that's a good thing. Designed to mimic bone, wood, and other natural materials, the porous objects are lighter than traditional products and can be strategically inserted into structures to provide higher stiffness in areas with high demand.
These porous structures, created by researchers at Princeton University and the Georgia Institute of Technology, feature spinodal microstructures — networks of specially designed holes that can be tuned to achieve optimized behavior at the macroscale. In a new study, published on March 16 in the journal Advanced Materials, the team combined different realizations of these spinodal microstructures to design and prototype facial implants for reconstructive surgery and stiff, lightweight parts for aircraft.
“There has been lots of work in recent years to optimally design structures and components for mechanical demands as well as to manufacture highly complex, biomimetic-architected materials at various scales, but integration of these two ideas is just beginning,” said Emily Sanders, a co-author and an assistant professor in the Woodruff School of Mechanical Engineering at Georgia Tech. “Here we make use of the mathematical underpinnings of spinodal architectures such that we can handle them almost effortlessly on both the design side and the manufacturing side, where connectivity of optimally-oriented mesoarchitectures has been a challenge.”
Davide Bigoni, a professor of solid and structural mechanics at the University of Trento who was not involved with the research, called the results a "breakthrough." He said: "The authors have found a clever way to allow a continuous transition between zones with different architectures. This is the ultimate concept of biomimicking, as all natural structures form continuous systems. This is a fact known since ancient times — 'natura non facit saltus,’ nature does not make jumps."
Many natural materials— including bone, animal horns, wood, and sand dollar skeletons — are full of holes. The empty spaces make the materials light, and in some cases allow body fluids to move through the pores. In bones, these spaces allow for a remodeling process that makes the bone more or less dense in response to physical demands. Creating synthetic materials with similar properties has been a challenge for engineers.
In the new study, the researchers mimicked these natural materials by designing microstructures with holes of different sizes, shapes, and orientations. The new objects are known as architected materials, which have customizable performance based on the relation of material and geometry. The holes can be shaped like spheres (like the ones in sand dollar skeletons), diamonds (bone), columns (wood), or lentils (horn). The researchers could infuse the material with stiffness in different directions by varying the shape. They controlled the material’s density by changing the holes’ size and altered the holes’ orientation within an object to increase stiffness in regions under strain.
"You have the actual structure and the microstructure working together to get superior performance, such as high stiffness with low weight," said Fernando Vasconcelos da Senhora, a graduate student at Georgia Tech and first author on the new study.
Research Methodology
To demonstrate potential uses, the researchers designed and 3D-printed a facial implant, such as the kind used to repair a major facial injury. Currently, surgeons use plastic or titanium to create porous implants that allow bone to regrow through holes, but these implants do not have the same tunability achievable with spinodal architectures. The researchers combined sections with column- and lentil-shaped holes to create an implant that was stiff enough to withstand the forces of chewing and had the right size holes to promote bone growth and healing. The prototype implant was made of a photopolymer resin, but it could be 3D printed using biocompatible materials for future use in patients.
The researchers said the technique opens the door to creating implants with many different types of material because the combination of geometry and material allows designers to fine tune performance.
“It’s not the base material that is better; it is the microscale features that are better,” said Sanders. “In theory, we could make the scaffolds out of any material – most appropriate would be to explore biocompatible materials.”
To show an entirely different application, the researchers combined three types of microstructures to construct a jet engine bracket — a critical part of an aircraft that holds the engine in place and must be both strong and lightweight.
"We have a technique that is quite powerful in the sense that it combines material architectures with optimization at different scales and its integration with additive manufacturing," said Glaucio Paulino, the Margareta E. Augustine Professor of Engineering at Princeton and principal investigator on the project. "It can have a broad range of applications in the sense that it is scales, so it can be applied in nano- and microtechnology, as well as at meso- and macroscales."
A key aspect of the materials' success is the seamless transitions from one type of microstructure to another within the same object. Abruptly switching between microstructures without connecting the network of pores would cause the material to split along the seams. Materials made with spinodal microstructures are also less likely to have weak spots because the holes occur randomly, instead of in regular patterns.
The team is already exploring additional applications for the microstructures. Currently, the technology is at the prototype stage, but they are eager to test out the properties of the materials more fully. "I'm interested in understanding the fundamental questions about how these architected materials behave," Sanders said.
Citation: Fernando V. Senhora, Emily D. Sanders, Glaucio H. Paulino. Optimally-Tailored Spinodal Architected Materials for Multiscale Design and Manufacturing. Advanced Materials. March 16, 2022. doi.org/10.1002/adma.202109304
Funding: The research article, Optimally-Tailored Spinodal Architected Materials for Multiscale Design and Manufacturing, was supported in part by funding from the National Science Foundation.
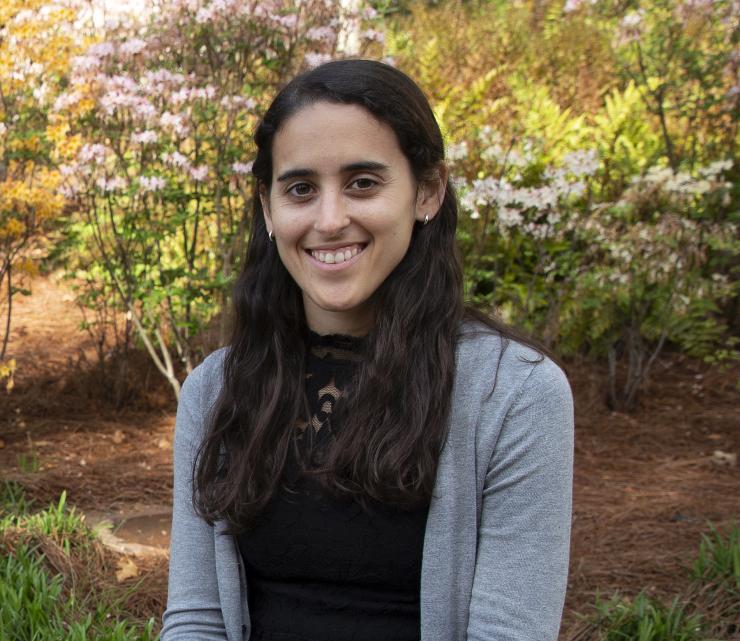
Emily D. Sanders, Assistant Professor, George W. Woodruff School of Mechanical Engineering
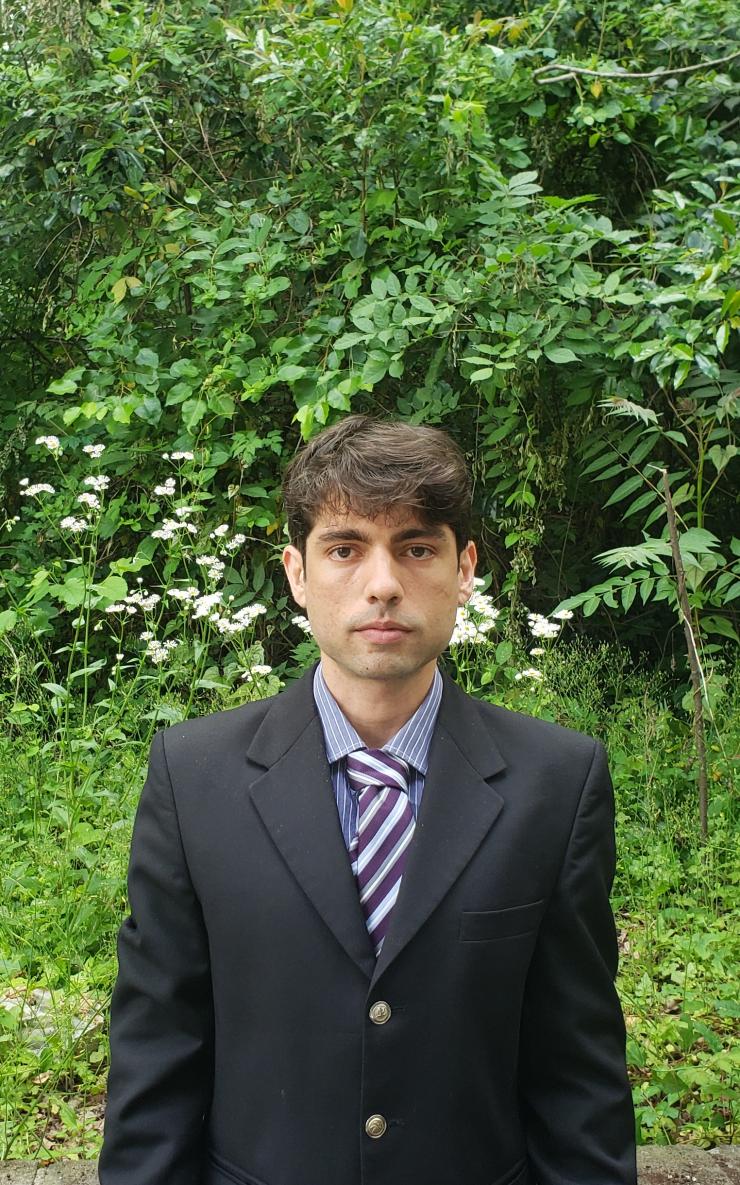
Fernando Vasconcelos da Senhora, Ph.D. Candidate, School of Civil and Environmental Engineering, Georgia Institute of Technology
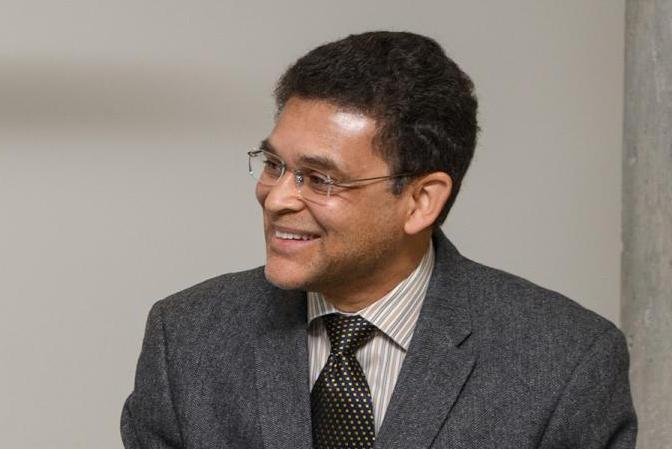
Margareta E. Augustine Professor of Engineering, Princeton University; Adjunct Professor, School of Civil and Environmental Engineering, Georgia Tech