Worms Inspire Wiggly Robots That Navigate All Landscapes
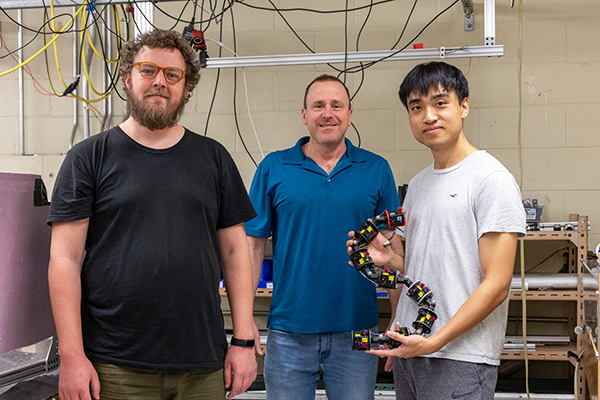
Worms and snakes seem to wiggle their way across varying environments without needing to learn the terrain. In more complex landscapes, they move even faster, using obstacles to propel themselves forward like a person pulling themselves up a ladder.
“They don’t alter their body-bending pattern no matter how dense the obstacles are,” said Tianyu Wang, a robotics Ph.D. student in the Institute for Robotics and Intelligent Machines and the George W. Woodruff School of Mechanical Engineering. “We were curious if this process was passively controlled, meaning they don’t have to ‘think’ about how to deal with obstacles — we consider this a kind of ‘mechanical intelligence.’”
To determine if this passive control hypothesis was correct, a team of roboticists, physicists, and engineers led by Daniel Goldman, the Dunn Family Professor in the School of Physics, and Hang Lu, professor and Cecil J. “Pete” Silas Chair in the School of Chemical and Biomolecular Engineering, developed a limbless robot. This robot helped them better understand the biology that makes worms and snakes so agile. The result is a robot that could be vital for missions in which humans and wheeled robots are limited, such as search and rescue, industrial maintenance, and planetary exploration.
“You can use the robot to confirm what you understand about biology,” said Christopher J. Pierce, a postdoctoral researcher in the School of Physics. “And at the same time, you might learn something from biology about how to build a better robot.”
The team published their findings in “Mechanical Intelligence Simplifies Control in Terrestrial Limbless Locomotion” in Science Robotics.
“You can use the robot to confirm what you understand about biology, and at the same time, you might learn something from biology about how to build a better robot.” —Christopher J. Pierce
Developing a New Limbless Robot
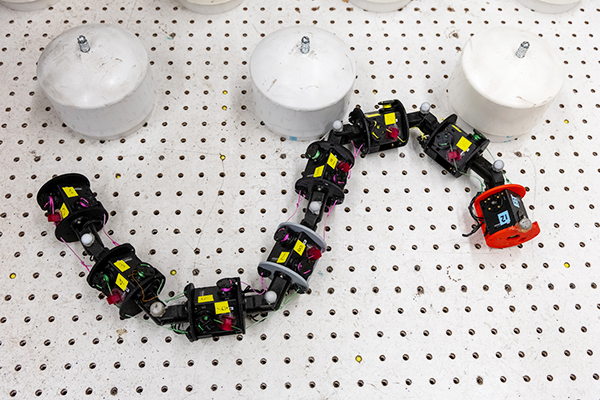
Snakelike, limbless robots have been in development for the last few decades, but previous versions relied on a chain of rotary motors connected by rigid links. The robot would sense what was in its environment. An algorithm would then tell it how to move, a process called computational intelligence, and the motors would precisely execute the algorithm’s instructions.
While these robots are impressive, they come nowhere near the flexibility of actual snakes and other limbless animals, like the nematode worm, C. elegans. Those nematodes are widely studied thanks to their see-through exterior that enables all 302 of their neurons to be visible under a microscope.
The researchers wanted to understand what made worms like C. elegans move more gracefully than their robotic counterparts. They used a biomechanical approach instead of the more typical emphasis on nervous system behavior. They posited that worms’ body material properties change as they move, using the elasticity and geometric arrangement of the muscles running along each side of the body; this means worms don’t need to engage their broader nervous system to handle all aspects of locomotion, a concept the researchers named “mechanical intelligence.”
“Our robot can handle a huge amount of unpredictability without having to use computational resources to deal with one crevice or large rock.” —Tianyu Wang
Mastering Mechanical Intelligence
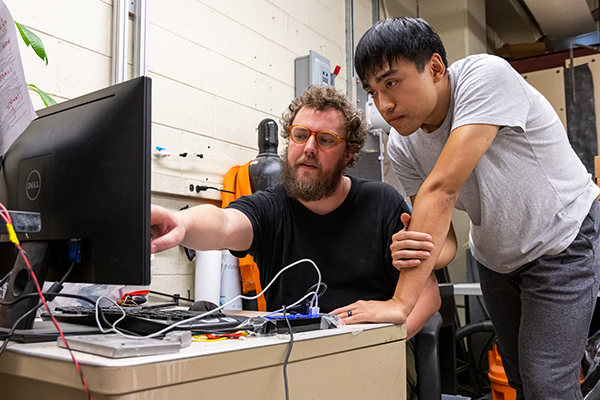
Applying this mechanical intelligence concept, the researchers built a robot that moved bilaterally with two independently controlled cables that could pull it from left to right. Just like a worm moves, when the cable is pulled one way, half of it tightens while the other half goes slack, enabling it to bend around objects. Unlike earlier snake robots that achieved bends with a single motor on each joint, this robot models the two bands of muscle found more commonly in nature.
“We used the robot as a simplified model to understand how biological locomotion works,” said Pierce. “The biological principles can help us build better control algorithms for these robots to move smoothly.”
To investigate the benefits of mechanical intelligence, the team built tiny obstacle courses and sent nematode worms through them to see how well they performed. They also sent the robot through a similar course and found that it moved through its course about as effectively as the real worms. They noticed that the worms made the same type of body movements when they collided with obstacles as the robot did.
These findings verified that mechanical intelligence alone is sufficient for a limbless robot to reproduce the movement of nematodes.
“Our robot can handle a huge amount of unpredictability without having to use computational resources to deal with one crevice or large rock,” said Wang. “If you can offload that computation for obstacles onto body mechanics, you free up onboard computational resources to use the computation to do more complex things.”
Instead of sensing the environment, the robots could use this computational intelligence to capture images, send communications, or perform other vital tasks.
Whether navigating an area affected by a natural disaster or a dense jungle, robots like these could usher in a new era of search and rescue and exploration. The possibilities are as fluid as the inspiration.
Writer & Media Contact: Tess Malone | tess.malone@gatech.edu
Photos & Video: Christopher McKenney