Faces of Research: Meet Jud Ready
Jul 07, 2022 — Atlanta, GA
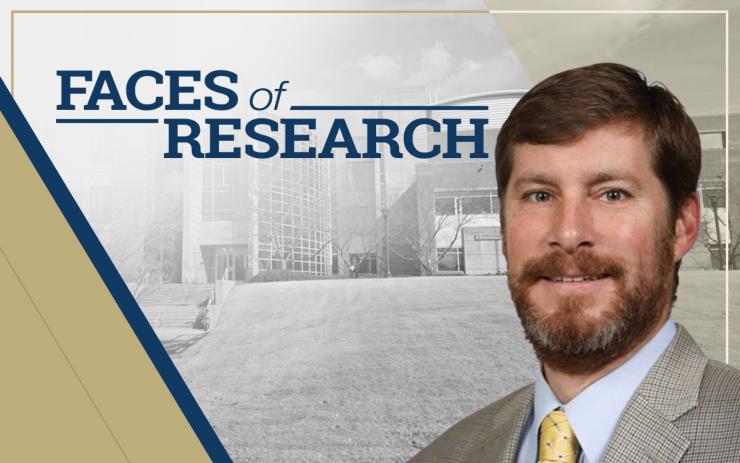
The Nano Group at the Georgia Tech Research Institute develops novel applications for carbon nanotubes and graphene based on their highly distinct electrical and physical properties. This group has developed thriving partnerships both in industry and government that led to research across a variety of ranging from functional fabrics and 2D materials to photovoltaics and supercapacitors.
This installment of the Faces of Research Q&A series is with Jud Ready, who leads the Nano group, is deputy director of innovation initiatives at Georgia Tech's Institute for Materials (IMat), and is an adjunct professor in the School of Materials Science & Engineering.
What is your field of expertise and why did you choose it?
I am a materials scientist. I applied for admission to Georgia Tech in 1989 for aerospace engineering. I was declined admission to that major, but was instead admitted to Tech as a "UEC" (undecided engineering candidate). A then-requirement of that "major" was to take three one-hour courses in three different majors during your freshman year to help you decide by your sophomore year what you wanted to do with your life. The three I chose were: civil engineering (CE), materials science engineering (MSE), and electrical engineering (EE). After AE, MSE most resonated with my interests of becoming an astronaut. Microgravity materials science was my interest at the time, but my interests evolved towards materials used in electronics, and ultimately my doctorate was on copper metallurgy (corrosion of printed wiring boards).
What makes Georgia Tech research institutes unique?
The "embedded" connection to the academic side of Georgia Tech gives us an unfair advantage over our competitors by having so many innovative academic resources (people, students, labs, etc.) right at our fingertips.
What impact is your research having on the world?
We are in the final stages of integrating a spacecraft that will map the water-ice resources on the moon: gtri.gatech.edu/newsroom/lunar-flashlight. We expect it to launch Dec. 27, 2022.
What do you like to do in your spare time when you are not working on your research or teaching?
I am a former Boy Scout and current scout leader. I enjoy camping, volunteerism, and community service. I am also a youth-league sports coach for my children and am now — after three kids — in my 13th season overall! Basketball is my favorite sport; I love to dribble the ball up and down the court. I am also an enthusiastic supporter of Georgia Tech athletics, and am an instrument-rated airplane and helicopter pilot.
Droplet Toolbox Opens New Possibilities for Genetic and Drug Screening of Small Animals
Jul 07, 2022 — Atlanta, GA

C. elegans, a freely living nematode that varies in size from a couple hundred microns at the larval stage to one millimeter once adult.
Genetic screens and drug screens play an essential role in the understanding of gene functions and the development of therapeutics. Genetic screens can identify genes contributing to a defect or disease state, while drug screens search for treatments that can restore normal function.
Traditionally, large-scale screens have been performed on single cells because of their small size and cost-efficiency, but with the limitation of missing the complexity of whole animals.
Screening animals allows for tackling phenotypes more relevant to human diseases, in particular dynamic phenotypes such as brain activity, muscle activity, or behavior. Such screens present huge benefits for the study of neuropsychological disorders and behavioral disorders. However, the challenge is that handling animals is far more challenging than handling single cells – especially when the need is to scale up to the thousands.
A team of researchers led by Hang Lu, a professor in Georgia Tech’s School of Chemical and Biomolecular Engineering, published a paper in the journal Small on a technological platform that would solve this bottleneck using droplet microfluidics.
Encapsulating microscopic animals in tiny droplets transposes the problem into a different perspective. Instead of manipulating animals directly, one now aims at handling a “passive” object containing an animal, Lu said. Working with droplets also has several advantages: no cross contamination, small volumes, and a gentle way of trapping and transporting the animal.
“Using droplet microfluidics allows for processing animals faster, user bias-free, and with nanoliter amounts of reagents. This platform is ideal to study the animal reactions to dynamic alterations of its chemical environment,” Lu said.
Studying C. elegans
As a proof of application, the team worked with C. elegans, a freely living nematode that varies in size from a couple hundred microns at the larval stage to one millimeter once adult. C. elegans is a widely used model organism in genetics that was first introduced in the 1960s. Several Nobel Prizes have been rewarded to researchers working on this model.
Besides having its genome fully sequenced and connectome fully determined, this mainly-hermaphroditic nematode has a large progeny, short reproduction cycle, and is inexpensive to maintain. Altogether, these attributes have established C. elegans as a key organism for large-scale screens. Traditionally, the screens have been performed manually and required months, if not years, of graduate student time and been often limited to somewhat simple animal responses.
The droplet platform may speed up that process as well as broaden the scope of assays performed on the animals. “We have developed a droplet toolbox that allows us to manipulate the animals and their environment and perform elaborate automated protocols” explained Guillaume Aubry, a research scientist in the Lu lab.
The team members first created an animal encapsulation system where each animal is packaged in an aqueous droplet. Then they created various droplet manipulators to alter the animal’s environment. In particular, a liquid exchanger allows researchers to swap the animal from one droplet to another. Finally, they established a method for programming protocols and performing tasks automatically.
Increased Throughput, Applications
The researchers demonstrated a throughput of more than a hundred animals per hour for cases of observing the animal behavior in response to a chemical stimulant. They also showed that this platform can be used to monitor brain activity simultaneously, which opens countless applications in neuroscience to decipher neuronal circuitry, dynamics, and correlate brain activity with behavior.
The team explained that the platform is adaptable to other small animals in the micro- to millimetric range. Because the design principle relies on droplets, the system is scalable to accommodate the animal size. As an example, the team scaled down the system to work with the first larval stage of C. elegans, which is considerably smaller, and demonstrated monitoring the larvae’s neuronal activity to a sudden change of chemical environment.
“Ultimately this platform will help to bridge the gap between environment, genotype, and phenotype,” Aubry said. “The platform opens up exciting possibilities in particular for the study of neurodevelopmental disorders. With this scalable tool, one can envision monitoring the development of single individuals and assessing their behavioral and neuronal activities over time. Another exciting avenue is to take further advantage of the nanoliter volumes to pursue drug screens, for example on the effect of neuronal activity.”
Funding
The authors acknowledge National Institute of Health (NIH R21NS117066, R01NS096581, and R01AG056436) for funding. This work was performed in part at the Georgia Tech Institute for Electronics and Nanotechnology, a member of the National Nanotechnology Coordinated Infrastructure (NNCI), which is supported by the National Science Foundation (Grant ECCS-1542174).
Citation
G. Aubry, M. Milisavljevic, and Hang Lu “Automated and Dynamic Control of Chemical Content in Droplets for Scalable Screens of Small Animals” Small 18, 2200319 (2022) DOI: 10.1002/smll.202200319 https://onlinelibrary.wiley.com/doi/full/10.1002/smll.202200319 (Supplemental Movie 5 and Supplemental Movie 7 of the Supporting Information of the paper)
Cleanroom User Spotlight: Alex Weidenbach
Jul 05, 2022 — Atlanta, GA

Alex Weidenbach is a graduate research assistant and Ph.D. student at Georgia Tech working with W. Alan Doolittle. In the following Q&A, Weidenbach briefly discusses his work in the IEN cleanroom and gives advice to current and future users.
How long have you been using the IEN Cleanroom?
I was hired as an intern at the Institute for Electronics and Nanotechnology (IEN) in 2014 during my undergraduate studies at Georgia Tech. In this role, I worked with both the processing and equipment teams and gained a wide range of skills in just about every aspect of cleanroom work. Those skills ultimately led to a job at Axion Biosystems, a local BioMEMS company, upon graduation. While at Axion, I continued to work in the inorganic cleanroom on proprietary research on microelectrode arrays (MEAs). I also developed some new fabrication processes to increase Axion’s manufacturing throughput. I returned to Georgia Tech to pursue my Ph.D., and I am currently in my fifth year of Ph.D. studies under Professor W. Alan Doolittle. All in all, I have been working in the inorganic cleanroom for the past seven years, and I have done everything from tool maintenance to consulting to academic research.
What tools do you use when you are in the cleanroom and what are you doing?
I primarily use the Denton Discovery 2 sputterer to co-deposit lithium-containing films to make memristive devices for neuromorphic computing applications. To make these films into devices, I frequently use a plethora of tools in the IEN cleanroom which includes the SCS G3P8 Spinner, Karl Suss TSA MA-6 Mask aligner, Heidelberg MLA 150, Vision RIE, Plasma Therm ICP, CtrLayer AET RTP, and the SSI RTP. I regularly need photolithography, dry etching, and rapid thermal annealing to finish my devices.
What is/has been your favorite project you have worked on in the IEN cleanroom?
My own research on memristive devices has been the most rewarding work I’ve done in the IEN cleanroom, though I’m not sure there has been a project that I did not enjoy. I find fabrication to be mentally satisfying and personally fulfilling, so I find enjoyment in the cleanroom work itself. I especially enjoy interacting with other users, recommending tools, and trying to help improve their process flows.
What advice do you have for people thinking about using a tool in the IEN cleanroom?
My advice to future cleanroom users is to make sure you get trained on multiple tools that can perform the same process. Having backup tools ready in case your favorite tool goes down or you run into issues is an absolute must, and it will save you countless hours in the future. Plan ahead and get trained on as many tools as possible. Also, take care to understand how the tool works and what exactly the tool is doing rather than just learning how to operate it. By knowing what is going on inside the chamber of the tool you are using you can more easily debug your process when you inevitably run into problems or challenges with your devices.
What is your favorite thing about the IEN Cleanroom?
My favorite things about the IEN cleanroom are the number of tool options and the amount of space available to quickly prototype new devices and explore fabrication processes. There are not many general-use cleanrooms set up to do what IEN does at the scope in which it operates. Having so many tools available really makes exploring new fabrication techniques and replicating research easier.
Growing Nanowires to Create Functional Devices for On-Demand Nanoelectronics
Jul 05, 2022 — Atlanta, GA

Michael Filler, associate director for research programs in Georgia Tech’s Institute for Electronics and Nanotechnology (IEN), was recently featured on the “Stories from the NNI” podcast. In the episode, Filler discusses balancing functionality and manufacturing scale during the process of making nanowires, his vision for on-demand nanoelectronics, and the benefits of interdisciplinary research.
When it comes to on-demand electronics, Filler and his team are working to develop a process that allows circuits to be built anywhere, without the need for expensive facilities.
“There are a lot of folks out there who have built on-demand platforms for circuit boards,” Filler explains. “But, in terms of nano, in terms of building chips in an on-demand fashion, we don’t have that technology.” Filler and his team are building a platform to do just that.
Listen to the entire podcast here.
Learn more about Filler’s work in the Filler Lab at Georgia Tech.
Georgia Tech Researchers Share Knowledge at 20th Hilton Head Workshop
Jul 01, 2022 — Atlanta, GA
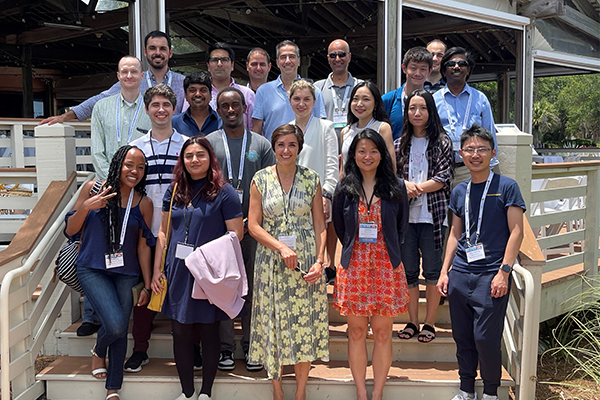
Hundreds of researchers gathered in Hilton Head, SC, from June 5-9, for the 2022 Hilton Head Solid-State Sensors, Actuators, and Microsystems Workshop. This was the 20th meeting of the biennial event, which is known as one of the premier workshops for researchers to discuss recent advances in microelectromechanical systems (MEMS).
The workshop started in 1984 and draws attendees from academia, industry, and government organizations with diverse backgrounds in engineering and science. It opened to international attendees in 2020 and provides a forum for knowledge exchange and collaboration. This year’s theme was "Preparation and Prevention: Tackling our Grand Challenges."
Nine papers from Georgia Tech researchers in the Institute for Electronics and Nanotechnology (IEN) were accepted to the conference. The paper, A Microtip Equipped Bidirectional Microrobot for Navigating on and Penetrating a Leaf Surface, presented by Tony H. Wang, Dea Gyu Kim, Zhijian Hao, and Azadeh Ansari, won the Springer Nature Best Poster Award. In addition, the paper Wafer-Level High-Aspect-Ratio Deep Reactive Ion Etching of 4h-Silicon Carbide on Insulator Substrates presented by Ardalan Lotfi, Micheal P. Hardin, Zhenming Liu, Alex Wood, Chris Bolton, Kevin Riddell, Huma Ashraf, Joanne Carpenter, and Farrokh Ayazi, was one of two runners up in the category.
“I consider this the flagship North American conference for MEMS researchers,” said IEN Executive Director Oliver Brand. “It is a highly interactive workshop and a great opportunity for our graduate students to learn about the newest research and mingle with their peers from the top MEMS research groups across the country.”
In addition to Georgia Tech’s strong presence in paper presentations, IEN Principal Research Scientist Paul Joseph ran a National Nanotechnology Coordinated Infrastructure (NNCI) booth to spread the word about the initiative, which the National Science Foundation funds. Ansari, Brand, Ayazi, and Nima Ghalichechian also served on the local organizing committee, and IEN sponsored the workshop.
The other papers presented at the workshop are listed below:
Power Handling Challenges of High KT2 AlScN Lamb Wave Resonators
Mingyo Park, Yue Zheng, and Azadeh Ansari
Exploiting Nonlinear Properties of VO2 in a mmWave Antenna-Coupled Sensor
Shangyi Chen, Mark Lust, and Nima Ghalichechian
A High-Q Solid Disk BAW Gyroscope in Monocrystalline 4h Silicon-Carbide with Sub-PPM As-Born Frequency Split
Zhenming Liu, Ardalan Lotfi, Michael P. Hardin, and Farrokh Ayazi
Utilization of Varying Transient Response Times in Gravimetric and Impedimetric Multivariate Gas Sensor with Single Polymeric Sensing Film for Enhanced Selectivity
Steven A. Schwartz, Luke A. Beardslee, and Oliver Brand
An Out-of-Plane Wide Bandwidth Micro-G FM Accelerometer with Differential Output
Seungyong Shin, Tanya Chauhan, Justin Matthews, Haoran Wen, and Farrokh Ayazi
Enhancement of Q and K2 in AL0.8SC0.2N/GAN/Sapphire Surface Acoustic Wave Resonators Using Semiconductor Ground Contact
Yue Zheng, Jialin Wang, Mingyo Park, Ping Wang, Ding Wang, Zetian Mi, and Azadeh Ansari
Piezoresistive Micro-Pillar Sensor for In-Plane Force Sensing for Biological Applications
Isha Lodhi, Durga Gajula, Devin K. Brown, Wilbur A. Lam, David R. Myers, and Oliver Brand
Nano@Tech Fall 2022 Series | Integrated Photonics
Integrated Photonics
Featuring Stephen Ralph | School of Electrical and Computer Engineering at Georgia Tech
Nano@Tech Fall 2022 Series | Thermally Responsive Materials for Clean Water and Energy
Thermally Responsive Materials for Clean Water and Energy
Featuring Akanksha Menon | School of Mechanical Engineering, Georgia Tech
Nano@Tech Fall 2022 Series | Ultrasound for Brain Imaging and Therapy
Ultrasound for Brain Imaging and Therapy
Featuring Chengzhi Shi | School of Mechanical Engineering, Georgia Institute of Technology
Nano@Tech Fall 2022 Series | Biomimetic Scaffolds for Tissue Repair and Regeneration
Biomimetic Scaffolds for Tissue Repair and Regeneration
Featuring Younan Xia | School of Biomedical Engineering at Georgia Tech and Emory University
Nano@Tech Fall 2022 Series | Next-Generation Vertical GaN Power Devices Using Selective-Area Doping Techniques
Next-Generation Vertical GaN Power Devices Using Selective-Area Doping Techniques
Featuring Spyros Pavlidis | School of Electrical and Computer Engineering, NC State University