Research Overview
Materials research at Georgia Tech is comprehensive, addressing the major technologies that can improve our lives in the next century and beyond. It ranges from advances in polymers and macromolecules, to nanostructures and materials for engineered devices, to materials and interfaces for catalysis and separations, to functional electrodes for batteries and fuel cells, to functional photonic and electronic materials, to advanced structural materials, to name a few.
Research Strengths
Materials and Interfaces for Catalysis
Advanced materials and interfaces (including surfaces, thin films, and membranes) are a key to developing the next generation of catalysts, separation processes, gas and liquid storage technologies, and environmental remediation methods. These materials and technologies are at the heart of industrial processes that generate several trillions of dollars per year of fuels, chemicals, polymers, industrial materials, and purified water.
However, these processes use large amounts of energy (about 15-20% of the world’s total consumption) and are increasingly challenged by their environmental footprint and the growing scarcity of fossil-based raw materials such as petroleum. An overarching grand challenge is the need for a new generation of advanced materials and interfaces for a host of sustainable chemical processes that provide renewable (or cleaner) fuels and industrial chemicals, inexpensive purified water, and clean air with low levels of greenhouse gases and pollutants.
Georgia Tech is a leader in this area, with a large and highly collaborative effort in cutting-edge fundamental and applied research to discover and develop polymeric, inorganic, and metal-organic materials, and devices based upon these materials, to impact ‘grand challenge’ problems. These include inexpensive CO2 capture, energy-efficient and high-performance catalysis and separations in the chemical sector, biofuel and biorenewable chemical production, efficient water purification and wastewater treatment, and processing of fuels and chemicals in harsh/toxic environments.
The DOE recently awarded a four-year $11.2 million grant to Georgia Tech to lead an EFRC that studies materials degradation caused by acid gases. Directed by Krista Walton, an Associate Professor in ChBE, the new center involves research teams from six universities and a government laboratory. Collaborating with Georgia Tech are researchers from Lehigh University, University of Alabama, University of Florida, University of Wisconsin, Washington University in St. Louis, and Oak Ridge National Laboratory.
Named the Center for Understanding and Control of Acid Gas-Induced Evolution of Materials for Energy (UNCAGE-ME), the Georgia Tech-led effort is one of 10 new EFRCs recently funded by the DOE.
Core capabilities include a comprehensive infrastructure for synthesis, structural and functional characterization, computational analysis, and application development for a large diversity of materials classes. These capabilities are sustained by strong industrial and federal research support.
Electrochemical Energy Storage and Conversion
Development of improved high-performance electrodes and electrolytes is critically important to the efficiency and overall performance of next generation energy storage and conversion applications, such as electrochemical capacitors, batteries and fuel cells for grid and distributed energy storage and conversion, electric and hybrid electric vehicles and ships, energy efficient industrial equipment and portable electronic devices.
For example, stable high capacity anodes and cathodes increase energy density of Li-ion batteries. Similarly, improvements in the electrode design for other types of batteries and supercapacitors will result in their faster charging, increased energy density, lower costs, and longer, safer operation. Further developments of coking- and contaminant-tolerant anodes for solid oxide fuel cells will make it possible for efficient and cost-effective conversion to electricity of a wide variety of fuels, including hydrogen, hydrocarbon fuels, coal gas, and bio-derived fuels. Similarly, the air-breathing cathodes critically influence the efficiency and power density of fuel cells and ultra-high energy metal-air batteries.
Energy and power density, rate capability, and cycling life of batteries, fuel cells, and supercapacitors depend critically on the structure, composition, morphology, and architecture of the electrode and electrolyte materials. However, quantifying and controlling the processing-structure-property relationships for these materials is extremely challenging.
Grand challenges facing the development of a new generation of energy storage and conversion systems include: (1) unraveling the mechanisms of surface and interfacial reactions relevant to chemical and energy transformation processes, (2) optimizing the rate of change and mass transport along surfaces, across interfaces, and through porous electrodes by design and control of mesoscale structure, (3) directing assembly of hierarchical functional materials with unique functionality,(4) understanding defect mesostructure and its effect on surface catalytic activities in fuel cells, (5) implementing predictive multiscale modeling and simulation of porous electrodes and their evolution under realistic operating conditions, and (6) expediting new materials discovery for applications by combining computation, modeling and experiments.
Faculty at Georgia Tech in MSE, ME, ChBE and other academic units have collectively developed some unique synthesis, processing, and fabrication of new electrode materials and novel structures of bio-inspired complexity and functionality. We also developed a number of advanced in situ characterization methods for materials and devices that employ campus equipment in addition to facilities at the national laboratories, such as in situ synchrotron X-ray diffraction for batteries. We have also developed various components for multiscale modeling and simulation of charge and mass transport relevant to chemical and energy transformation processes over a wide range of length and time scales. Finally, we have developed some unique in-situ and in-operando characterization of structure, composition, and morphology of electrode materials.
Structural Materials
Light weighting vehicles, infrastructure, and products not only maintain or even enhance performance, but promise to positively impact energy sustainability with numerous potential applications in both defense and civilian sectors. Consequently, the design and production of lightweight metals and reinforced composites with superior combinations of properties in the finished product are of utmost importance, particularly in the transportation and energy sectors. Georgia Tech faculty employ an integrated approach that brings together expertise in materials science, manufacturing, product design, systems engineering, and data/information sciences to address this challenge. Capabilities include:
The Mechanical Properties Research Laboratory at Georgia Tech is a leading US university laboratory in experimental studies of deformation, fracture and fatigue of structural materials, as well as coupling such experiments with mulitiscale modeling and simulation.
- A broad suite of experimental facilities and customized protocols that allow high throughput acquisition of multiscale multi-modal measurements (e.g., microscopy, orientation image mapping, indentation, strain mapping, residual stress measurements, non-destructive imaging techniques).
- A broad suite of experimental facilities for manufacture of polymer composites including a unique custom-made SMC production line which allows for high throughput manufacture of SMC composites at small scale but of industrial specifications that can produce composites that cover a large design space in terms of material composition and micro-structure and processing conditions.
- A broad suite of multi-physics multiscale modeling approaches that span a wide range of length and time scales (e.g., atomistics, dislocation dynamics, phase-field, Monte-Carlo, crystal plasticity, finite element).
- Novel analytic tools for extracting property-structure-property linkages from large experimental and simulation datasets and presenting them in forms that are easily accessible by design tools.
Organic and Inorganic Photonics and Electronics
Organic-based materials for photonics and electronics The Center for Organic Photonics and Electronics (COPE) was founded at the Georgia Institute of Technology in 2003 to foster research, education, and innovation in the field of organic photonics and electronics. The ability to produce lower-cost, flexible, wearable, and eco-friendly electronic applications – such as lighting, diagnostic devices, screens, and batteries – using various organic-based materials has great potential as the world moves toward greater sustainability in products, processes, and resources. COPE’s research ranges from materials theory and modeling to device design and fabrication. Applications include:
- Organic materials and device architectures for future solid-state lighting and photovoltaics applications
- Nanocomposites for high energy-density storage applications • Organic and hybrid thin-film transistors for flexible electronics applications
- Novel nanolithography techniques for the development of new electronics surfaces
- Barrier coatings for the packaging of organic and printed electronics
- Organic materials for all-optical switching and computing
Printed electronics offers a paradigm change in manufacturing, cost and environmental impact when compared to the conventional semiconductor industry. Printed electronic devices are expected to be mass-produced from less-energy-demanding roll-to-roll processes over large areas and on flexible substrates with techniques that closely resemble the well-known mass production of printed media on paper. Plastic electronics markets are expected to show strong growth in the next decade to reach a value of $80 billion by 2023.
This massive projected market growth offers a great economic opportunity but also introduces new challenges. Currently flexible plastic substrates continue to be considered the natural choice for developing printed electronic applications. Conventional flexible substrates suffer from some mechanical limitations, including some residual built-in stress that is introduced during the manufacturing process used to fabricate these films, for example extrusion.
To realize the full potential of organic printed electronics, new substrates based on multifunctional materials need to be developed. When considering the evolutionary trend of organic printed electronics it becomes apparent that the substrate platform is moving from being just flexible towards becoming increasingly conformal, stretchable, and soft. Substrates for printed electronics will also have to become more compatible with the human body. Ultimately, new material concepts will need to be explored to develop biocompatible devices for in-vivo electronics that can be implanted into the human body while minimizing biological rejection and inflammation.
Inorganic compound semiconductors
Wide bandgap semiconductors based on GaN and ternary alloys of AlGaN and InGaN are poised to play a critical role the future of electronics. Unlike Si, these materials can have bandgaps that are tunable with material composition over a range of 0.7 to 5 eV. This factor allows them to be used for optoelectronic devices that are the basis of the solid-state lighting revolution which is posed to save billions of dollars in reduced energy demand while lowering harmful greenhouse emissions, solar cells that provide alternative green energy sources and the development of UV light sources for water purification and biological detectors.
The high carrier mobilities and large breakdown fields in GaN-based semiconductors allow them to be used in advanced RF communication systems operating at high frequency (tens of GHz) and high power. These devices will be used in advanced radar systems as well as broadband telecommunications enabling video on demand and other culturally transformative technologies. Additionally, the high voltages these devices can operate under (tens of kV) make them suitable for use in power electronic modules necessary for hybrid and electric vehicles, solar photovoltaic inverters, power supply miniaturization and efficiency improvements and smart grid applications. Combined with solid-state lighting, GaN is poised to play a significant role in clean energy technologies involved with electrical energy generation or conservation in our nation’s future.
To achieve the goal of doubling America’s energy productivity by 2030, significant research still remains in the development of GaN electronics and the Georgia Institute of Technology is ready to play a leading role. Improvement in growth quality and doping of GaN is needed to improve the performance of optoelectronics for solid-state lighting. Since lattice matched growth substrates for GaN remain a challenge, the control of growth defects on lattice mismatched substrates and the resistance to heat dissipation must be addressed.
Researchers at the Georgia Institute of Technology are developing methods based on molecular beam epitaxy and metal organic chemical vapor deposition to create improved materials and doping methods. These technologies have recently shown increases in certain key figures of merit related to the ability to carry electricity of over 40 times what was previously considered a practical limit and have greatly lowered defect densities in these crucial materials using newly developed technologies from the Georgia Institute of Technology. Researchers are also developing advanced thermal management solutions involving new thermal interface materials based on nanotechnology and active cooling solutions embedded in the device to improve reliability and efficiency.
Georgia Tech also has leading expertise in the metrology of the temperature and stresses in GaN electronics to verify device performance and yield new insight into device reliability. The Georgia Institute of Technology is home of the National Electric Energy Testing and Research Applications Center which is focused on solving problems energy transmission and distribution for utilities which will benefit greatly from new power electronics for the smart grid.
Overall, Georgia Tech has a strong history of leadership in the field and collaborative work with industry and governmental agencies in the area of GaN electronics and is poised to continue to make groundbreaking contributions in the future.
Collaborate with IMat
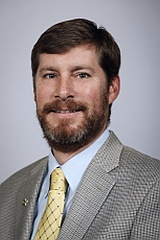
If you are looking to collaborate with one of our ground-breaking faculty or Strategic Investment Centers, please reach out to IMat’s Deputy Director of Innovation Initiatives.
Jud Ready
Deputy Director, Innovation Initiatives
Principal Research Engineer, GTRI
jud.ready@gtri.gatech.edu
404.407.6036
Additional Resources
Electrochemical Energy Storage & Conversion
- www.fcbt.mse.gatech.edu/liu.htm
- www.nano-tech.gatech.edu/
- www.nesac.gatech.edu
- www.energytech.gatech.edu
- www.hlchen.gatech.edu/people
- www.mtmcdowell.gatech.edu/index.html
Structural Materials
Organic and Inorganic Photonics & Electronics