Rattling Physics with New Math
Oct 21, 2024 —

A pair of Smarticle robots from the lab of Prof. Dan Goldman. Earlier research from his group observed the arise of order in active matter from the physics of low rattling. (Photo Credit: Christa M. Ernst)
If you’ve ever watched a large flock of birds on the wing, moving across the sky like a cloud with various shapes and directional changes appearing from seeming chaos, or the maneuvers of an ant colony forming bridges and rafts to escape floods, you’ve been observing what scientists call self-organization. What may not be as obvious is that self-organization occurs throughout the natural world, including bacterial colonies, protein complexes, and hybrid materials. Understanding and predicting self-organization, especially in systems that are out of equilibrium, like living things, is an enduring goal of statistical physics.
This goal is the motivation behind a recently introduced principle of physics called rattling, which posits that systems with sufficiently “messy” dynamics organize into what researchers refer to as low rattling states. Although the principle has proved accurate for systems of robot swarms, it has been too vague to be more broadly tested, and it has been unclear exactly why it works and to what other systems it should apply.
Dana Randall, a professor in the School of Computer Science, and Jacob Calvert, a postdoctoral fellow at the Institute for Data Engineering and Science, have formulated a theory of rattling that answers these fundamental questions. Their paper, “A Local-Global Principle for Nonequilibrium Steady States,” published last week in Proceedings of the National Academy of Sciences, characterizes how rattling is related to the amount of time that a system spends in a state. Their theory further identifies the classes of systems for which rattling explains self-organization.
When we first heard about rattling from physicists, it was very hard to believe it could be true. Our work grew out of a desire to understand it ourselves. We found that the idea at its core is surprisingly simple and holds even more broadly than the physicists guessed.
Dana Randall Professor, School of Computer Science & Adjunct Professor, School of Mathematics
Georgia Institute of Technology
Beyond its basic scientific importance, the work can be put to immediate use to analyze models of phenomena across scientific domains. Additionally, experimentalists seeking organization within a nonequilibrium system may be able to induce low rattling states to achieve their desired goal. The duo thinks the work will be valuable in designing microparticles, robotic swarms, and new materials. It may also provide new ways to analyze and predict collective behaviors in biological systems at the micro and nanoscale.
The preceding material is based on work supported by the Army Research Office under award ARO MURI Award W911NF-19-1-0233 and by the National Science Foundation under grant CCF-2106687. Any opinions, findings, and conclusions or recommendations expressed in this material are those of the authors and do not necessarily reflect the views of the sponsoring agencies.
Jacob Calvert and Dana Randall. A local-global principle for nonequilibrium steady states. Proceedings of the National Academy of Sciences, 121(42):e2411731121, 2024.
Undergraduate Anu Iyer Leads Parkinson’s Research Study
Aug 15, 2024 —

Iyer completed much of her research while in high school and submitted the paper for publication as a Georgia Tech first-year.
Anu Iyer, a Georgia Tech Dean’s Scholar, published her first research article as a first-year student — based on research conducted while she was in high school. She is the lead co-author of the paper published in Scientific Reports, a Nature Portfolio journal.
Iyer, now a second-year undergraduate majoring in biology with a pre-med focus, worked with researchers at the University of Arkansas for Medical Sciences (UAMS) to develop a novel voice-based diagnostic tool for Parkinson’s disease (PD).
“Essentially, we proved the feasibility of a telemedicine approach towards detecting PD,” says Iyer. “Through a three-second phone call, our machine-learning model recognizes patterns in data to detect Parkinson’s with a 97 percent accuracy rate.”
Iyer states that additional strengths of the project include the potential for detecting PD at an early stage, leading to improved treatment outcomes, and the practical benefits of a virtual diagnostic tool.
“Parkinson’s disease is a nervous system disorder that primarily affects the elderly population, and one of the many issues with detection is that symptoms must be analyzed in person,” explains Iyer. “In Arkansas, 75 percent of our population resides in medically underserved areas — it can be hard for them to access health facilities. Our research addresses the need for convenient detection via telemedicine.”
From science fairs to academic researcher
Iyer’s teachers at her STEM middle school encouraged her passion for science and discovery. A science fair enthusiast, Iyer led a sixth-grade team to win the state title for the Verizon Innovative Learning app, creating a smartphone app that turns off text notifications when a car reaches more than five miles per hour.
Iyer credits her middle school teachers for inspiring her to seek answers beyond what she found in her textbooks. During the summer between eighth and ninth grade, Iyer watched YouTube videos to teach herself machine learning, appreciating the opportunity to use artificial intelligence to analyze data and make predictions.
“Machine learning fascinates me because it holds so much potential,” says Iyer. “I've always been interested in computer science, but machine learning opened my eyes to new possibilities and taught me that I can pay it forward through applied bioinformatics.”
In ninth grade, she emailed UAMS professors with a research idea incorporating medicine and computer science. Her outreach led to a post as an undergraduate researcher, helping create a computer algorithm to detect eye disease. While working on a diagnostic AI model for malignancy, she began collaborating with Fred Prior, the chair of Bioinformatics at UAMS, who became a valued mentor.
“Dr. Prior introduced me to the joys of research and how small changes can make a big difference in our world,” says Iyer.
Prior assigned her to the team focusing on Parkinson’s in her 11th grade year — and she soon began taking on more of an active leadership role in the research. She spent the rest of high school juggling coursework with constructing code and drafting proposals to create the computer algorithm capable of detecting PD.
Progress and service
Iyer’s desire to improve the world through research led her to Georgia Tech.
“One thing that spoke to me is the Progress and Service motto,” says Iyer. “My career goals include becoming an empathetic researcher focused on reducing healthcare disparities. Specifically, I hope to specialize in developing diagnostic tools that are affordable and available for underserved areas.”
As lead co-author of the PD research study, Iyer spent much of her first year working with Prior and UAMS, participating in Zoom calls every Saturday. As a second-year, Iyer intends to continue working with UAMS on PD and machine-learning research. She has also taken on a new role as multiple principal investigator for a study related to chronic back pain management.
Lainie Pomerleau, who taught Iyer’s first-year English course, and is now an assistant professor of English at the College of Coastal Georgia, helped Iyer prepare the PD paper for publication. “Anu embodies Georgia Tech's mission to develop leaders who advance technology to improve the human condition,” says Pomerleau.
Despite her busy schedule, Iyer has immersed herself in the Georgia Tech community. She loves the climbing wall at the Campus Recreation Center and points to Cognitive Psychology as her favorite class. Iyer considers Explore, the science-centered living and learning community, to be one of the highlights of her first year.
“I really enjoyed being a part of Explore, living with other students who prioritize science,” says Iyer. “It was easy to make friends because we all had similar classes.”
In the spring of her first year, she was selected as a College of Sciences Ambassador, accompanying prospective students and their parents to science-related courses and answering their questions about campus life.
She plans to get more involved with researchers at Georgia Tech.
“I am a biology major, but one amazing thing about Georgia Tech is that there is a lot of encouragement to join labs outside of your major and pursue your interests,” says Iyer. “I’d like to work in a Georgia Tech lab, particularly in neurology.”
Looking forward to her next few years at the Institute, she’s excited about the possibilities ahead:
“Georgia Tech is well known for groundbreaking research,” she says. “I want to take advantage of Tech’s many opportunities — and fulfill my ultimate goal of making a positive impact in the world.”
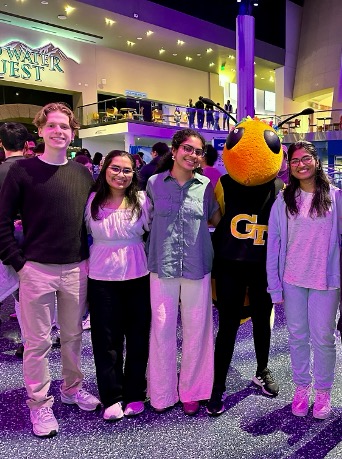
As a first-year, Iyer enjoyed diving into Tech's many events and activities, such as Georgia Tech Night at the Aquarium.
Laura S. Smith
Communications Officer II
College of Sciences
RBI Initiative Lead Profile: Will Gutekunst
Jul 30, 2024 — Atlanta, GA

Will Gutekunst
Will Gutekunst, associate professor in the School of Chemistry and Biochemistry at Georgia Tech, co-leads the interface of polymer science and wood-based materials initiative along with Blair Brettmann at the Renewable Bioproducts Institute (RBI). Gutekunst’s research explores the design of novel monomers for the design of recyclable polymers for a circular economy, fluxional materials, and 3D-printable ceramics.
Below is a brief Q&A with Gutekunst where he discusses his research focus areas and how they influence the interface of polymer science and wood-based materials initiative at Georgia Tech.
- What is your field of expertise and at what point in your life did you first become interested in this area?
My graduate training is in synthetic organic chemistry, and I focused on basic science problems at that time. Toward the end of my Ph.D., I became interested in applying my skill set to new research directions that could have a more direct impact on society. This led me to pursue postdoctoral research in polymer chemistry, which has been a source of inspiration ever since.
- What questions or challenges sparked your current renewable bioproducts research? What are the big issues facing your research area right now?
My first project in this space was initiated shortly after I arrived at Georgia Tech through RBI funding opportunities, and it has continued to be a theme ever since. One of the critical problems in my research is identifying monomers that can polymerize and depolymerize on command. This involves balancing the driving force of polymerization (enthalpy) with the unfavorable process of confining multiple monomers to a single chain (entropy). While we are making considerable progress in engineering appropriate polymerization enthalpies into monomers, the entropic side of the problem remains a significant challenge.
- What interests you the most in leading the research initiative on the interface of polymer science and wood-based materials? Why is your initiative important to the development of Georgia Tech’s renewable bioproducts research strategy?
The most exciting aspect of the initiative is the ability to bring together multiple strengths of Georgia Tech to work on a central goal. Solving problems at this interface involves the collaborative efforts of researchers in chemistry, processing, separations, and even data science. Identifying and gathering synergistic teams is critical to address this problem and additional goals in renewable bioproducts.
- What are the broader global and social benefits of the research you and your team conduct on the interface of polymer science and wood-based materials?
The goal of this research is to develop materials that are more recyclable and are derived from abundant feedstocks, which are two big problems rolled into one. The eventual product of this research will be access to materials that are more compatible with the environment while also drastically reducing the waste output of society.
- What are your plans for engaging a wider Georgia Tech faculty pool with the broader renewable bioproducts community?
Through the merger of the Georgia Tech Polymer Network with RBI, we can start to forge collaborations across a broader swath of the Georgia Tech community. This includes the organization of workshops, making connections between different student groups, and the development of center grants to tackle grand challenges in the field.
- What are your hobbies?
In my free time, I enjoy reading (non-science), pottery, and hiking.
- Who has influenced you the most?
My Ph.D. advisor (Phil Baran) and my postdoctoral advisor (Craig Hawker) both stand out in their impact on my scientific career. Through their guidance, I learned how to properly think about science and to always look ahead for the next big problem.
Priya Devarajan | RBI Communications Manager
Winners of the Seed Grant Challenge for Climate Solutions Announced
Jul 15, 2024 — Atlanta, GA
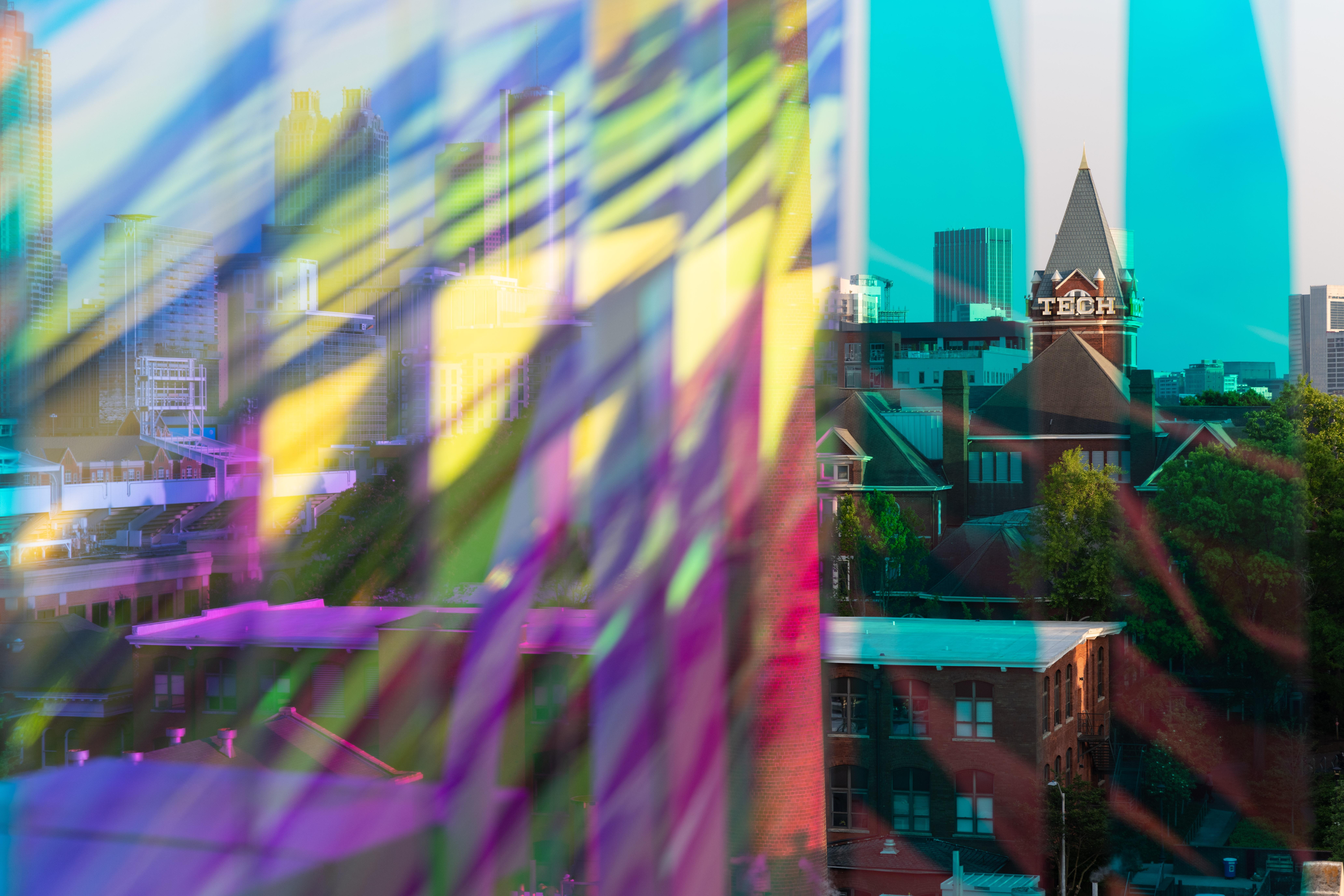
A view of Tech Tower from Crosland Tower. Photo: Georgia Tech
Nine early-career professors will pursue cutting-edge climate mitigation research during the upcoming year as part of the Seed Grant Challenge for Climate Solutions created by the Strategic Energy Institute (SEI) and the Brook Byers Institute for Sustainable Systems (BBISS).
Launched in April during the Frontiers in Science: Climate Action Conference and Symposium, the Challenge “provides seed funding for climate mitigation and adaptation research led by ambitious early-career faculty eager to work across disciplines,” explains Beril Toktay, Regents’ Professor and interim executive director of BBISS.
One goal of the Challenge is to facilitate research collaboration across the Institute. “Transitioning to a sustainable, clean energy system requires concerted collaboration across diverse disciplines,” says Tim Lieuwen, Regents’ Professor, David S. Lewis, Jr. Chair, and executive director of SEI. “Initiatives like this are instrumental in paving the way for such groundbreaking interdisciplinary work.”
The four selected proposals include researchers from five different schools and two centers, and will investigate biodiversity, coral reef resilience, lithium-ion battery recycling, and coastal resilience. “I am pleased with the range of proposals submitted by our assistant professors,” adds Susan Lozier, dean of the College of Sciences and Betsy Middleton and John Clark Sutherland Chair and professor in the School of Earth and Atmospheric Sciences. “Each proposal represents an opportunity to combine expertise from across the Institute to deepen our understanding of climate challenges and uncover possible solutions.”
Each of the following projects will receive a $15,000 seed grant to be used during the 2025 fiscal year:
Climate Solutions in the Most Biodiverse Regions on Earth: Testing Whether Warming Temperatures have set in Motion an “Escalator to Survival” in Tropical Rainforests
- Benjamin Freeman, assistant professor in the School of Biological Sciences
- James Stroud, assistant professor in the School of Biological Sciences
- Saad Bhamla, assistant professor in the School of Chemical and Biomolecular Engineering
- Amirali Aghazadeh, assistant professor in the School of Electrical and Computer Engineering
The research team seeks to test the “escalator to survival” concept, which theorizes that lowland tropical species will only be able to persist in the face of rising temperatures if they are able to shift their ranges to nearby foothills and mountains, where temperatures remain cooler.
Macro- and Microscale Drivers of Coral Reef Resilience in a Changing Climate
- Isaiah W. Bolden, assistant professor in the School of Earth and Atmospheric Sciences
- Lauren Speare, assistant professor in the School of Biological Sciences and the Center for Microbial Dynamics and Infection
The research team will develop transformative tools to evaluate reef health and resilience; detect impending compositional changes; determine the capacity for reef regeneration; and elevate mitigation strategies that preserve reef diversity and ecosystem services.
A Workforce and Community-Engaged Team Building Approach for Lithium-Ion Battery Recycling in the U.S. Southeast: Addressing Social and Ecological Implications
- Joe F. Bozeman III, assistant professor in the School of Civil and Environmental Engineering and the School of Public Policy
- Jennifer Hirsch, senior director of the Center for Sustainable Communities Research and Education
This project will build a transdisciplinary team to determine how to effectively unite community stakeholders, industry, social scientists, and engineers when applying for external grants to equitably establish a U.S. southeastern hub for EV-battery lithium recycling.
Building Coastal Resilience: Science-based Adaptive Solutions to Mitigate Hurricane-Induced Compound Flooding in the Southeast U.S.
- Ali Sarhadi, assistant professor in the School of Earth and Atmospheric Sciences
This project will quantify the risks associated with hurricane-induced compound flooding in a warming climate by developing physics-based hydrodynamic and AI models. The project aims to promote geographic equity in climate resilience and develop science-based, cost-effective adaptation strategies through active community engagement in Savannah, Georgia and Jacksonville, Florida.
Writer: Lindsay C. Vidal
2024 Frontiers in Science: Climate Action
Apr 22, 2024 — Atlanta, GA

Susan Lozier, Julia Kubanek, L. Beril Toktay, and Tim Lieuwen
This Earth Month more than 100 campus and community stakeholders gathered near the Georgia Tech EcoCommons for the 2024 Frontiers in Science: Climate Action Conference and Symposium.
On April 18, the College of Sciences hosted more than 20 speakers and panelists from across the Institute and Atlanta community presenting groundbreaking research and discussing innovations and ideas in climate change, challenges, and solutions.
Georgia Tech President Ángel Cabrera (M.S. PSY 1993, Ph.D. PSY 1995) kicked off the morning sessions by highlighting the Institute’s new Climate Action Plan, which outlines the pathway to achieve net-zero emissions by 2050. Cabrera’s remarks focused on Georgia Tech’s role on the frontlines of research and education informing how we respond to climate challenges — and noted that the Institute’s work must extend beyond our laboratories and classrooms.
“It is essential that we not only do the science, but that we also tell that science to the world,” Cabrera says.
Interdisciplinary inquiry
This year, Frontiers in Science featured an array of climate research and initiatives led by the College of Sciences, fellow colleges across Georgia Tech, and the wider Atlanta community.
Following a three-year hiatus of the Frontiers series, the 2024 edition re-envisioned the signature annual event as a research conference and symposium to convene campus experts — and to incubate seed grant proposals to support the work of early career faculty.
Frontiers previously hosted Nobel laureates and invited thought leaders for individual talks across the College’s six schools, and celebrated milestones like the International Year of the Periodic Table of the Chemical Elements.
“This year, we wanted to showcase what we are doing right here in the College of Sciences and throughout the Institute,” says Susan Lozier, dean of the College of Sciences, Betsy Middleton and John Clark Sutherland Chair and professor in the School of Earth and Atmospheric Sciences. “Our faculty are at the forefront of broadening our knowledgebase and uncovering solutions in areas critical to the planet and our well-being. We wanted to uplift that work and see what sort of connections could be made.”
Connections and collaboration were key themes of the day as faculty, staff, students, and alumni participants representing all six Georgia Tech colleges shared research results and ongoing work and discussed collaborative ideas for horizons ahead.
“Scientists alone cannot [create accurate models],” noted Annalisa Bracco, professor in the School of Earth and Atmospheric Sciences and associate chair for Research, who shared her own research alongside Lozier, who presented a version of her 2024 TED Talk on ocean overturning. “Engineers alone cannot do it. We need social scientists, policy makers, communicators.”
The importance of an interdisciplinary approach was reinforced by the Strategic Energy Institute at Georgia Tech (SEI) and Brook Byers Institute for Sustainable Systems (BBISS), which announced an interdisciplinary seed grant funding opportunity for assistant professors with ideas for new climate solutions.
Frontiers in focus
Across three themed sessions, faculty and leadership from the Colleges of Sciences, Engineering, and Design spearheaded talks on the ocean and cryosphere, biodiversity, carbon cycling, coastal wetlands, biofuels production, and beyond.
Panels on climate challenges across community, technological, and policy initiatives were hosted by Georgia Tech Vice President for Interdisciplinary Research and Professor in the School of Biological Sciences and the School of Chemistry and Biochemistry Julia Kubanek.
Following a networking lunch with climate table topics, Georgia Tech Executive Vice President for Research and Professor in the School of Electrical and Computer Engineering Chaouki T. Abdallah (M.S. ECE 1982, Ph.D. ECE 1988) kicked off the afternoon sessions — which also announced the scholarship recipients of a student video competition and featured videos with a pair of alumnae working in meteorology, climate research, and policy.
Afternoon highlights also included discussions on the Georgia Tech Climate Action Plan and Sustainability Next initiative, led by Jennifer Chirico (B.S. MGMT 1997, Ph.D. PUBP 2011), associate vice president of Sustainability for Georgia Tech Infrastructure and Sustainability, and Jennifer Leavey (B.S. CHEM 1995), assistant dean for Faculty Mentoring in the College of Sciences and interim assistant director for Interdisciplinary Education in the Brook Byers Institute for Sustainable Systems.
Although many of the presentations provided a stern outlook of the state of our ecosystems, the conference concluded with a sense of hope. This optimism was grounded in the range of opportunities that exist to address climate challenges — thanks, in part, to the body of knowledge and solutions being tested and explored by Georgia Tech researchers.
At the end of the day, Katie Griffin, a first year undergraduate student in Environmental Science, read Amanda Gorman’s poem Earthrise and provided this reminder:
All of us bring light to exciting solutions never tried before
For it is our hope that implores us, at our uncompromising core,
To keep rising up for an earth more than worth fighting for.
Experience the event in pictures with the College of Sciences’ Flickr account, and discover the highlights through the day’s live tweets on College of Sciences’ X account.
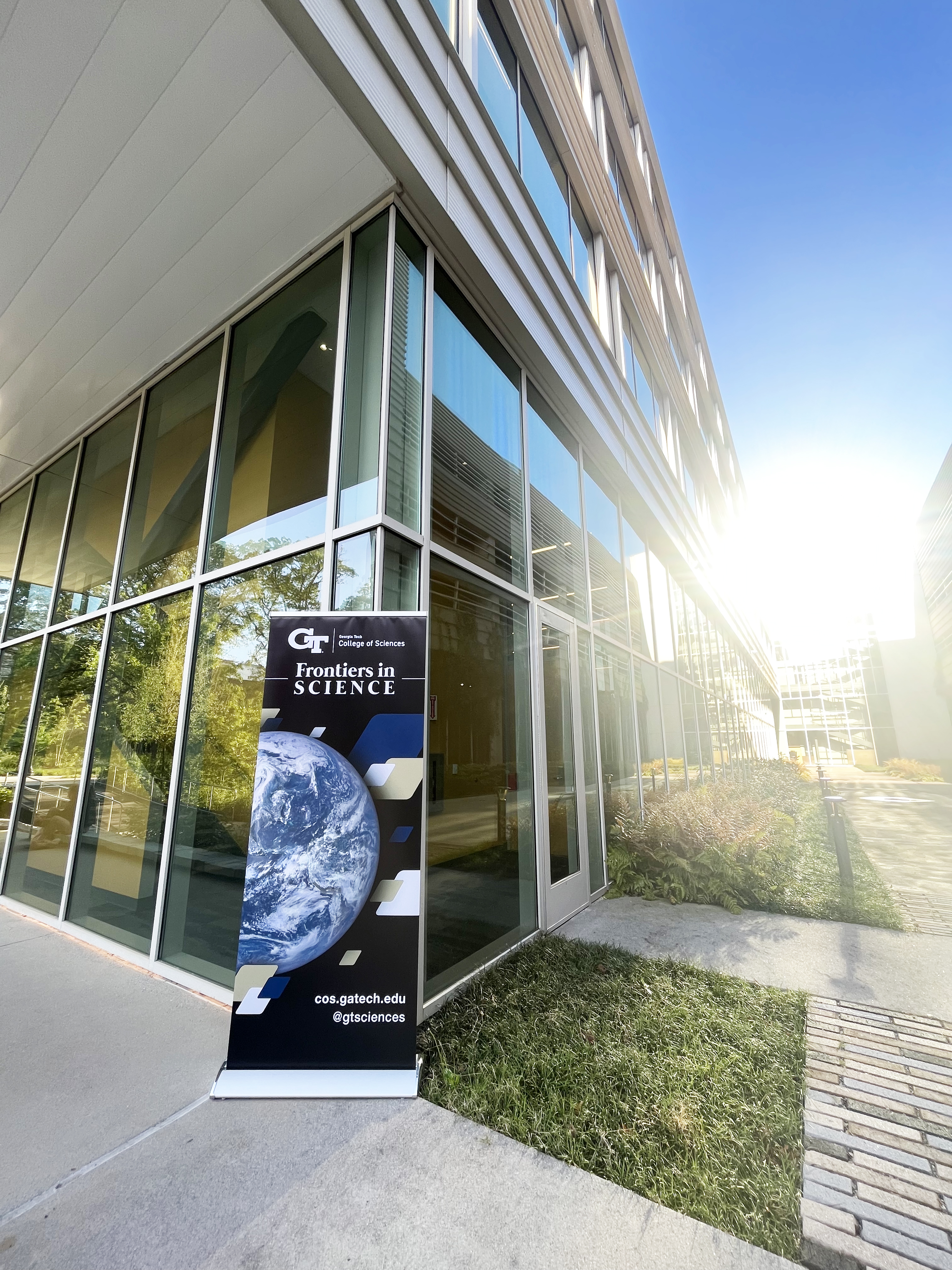
Frontiers in Science Banner Outside at Sunrise
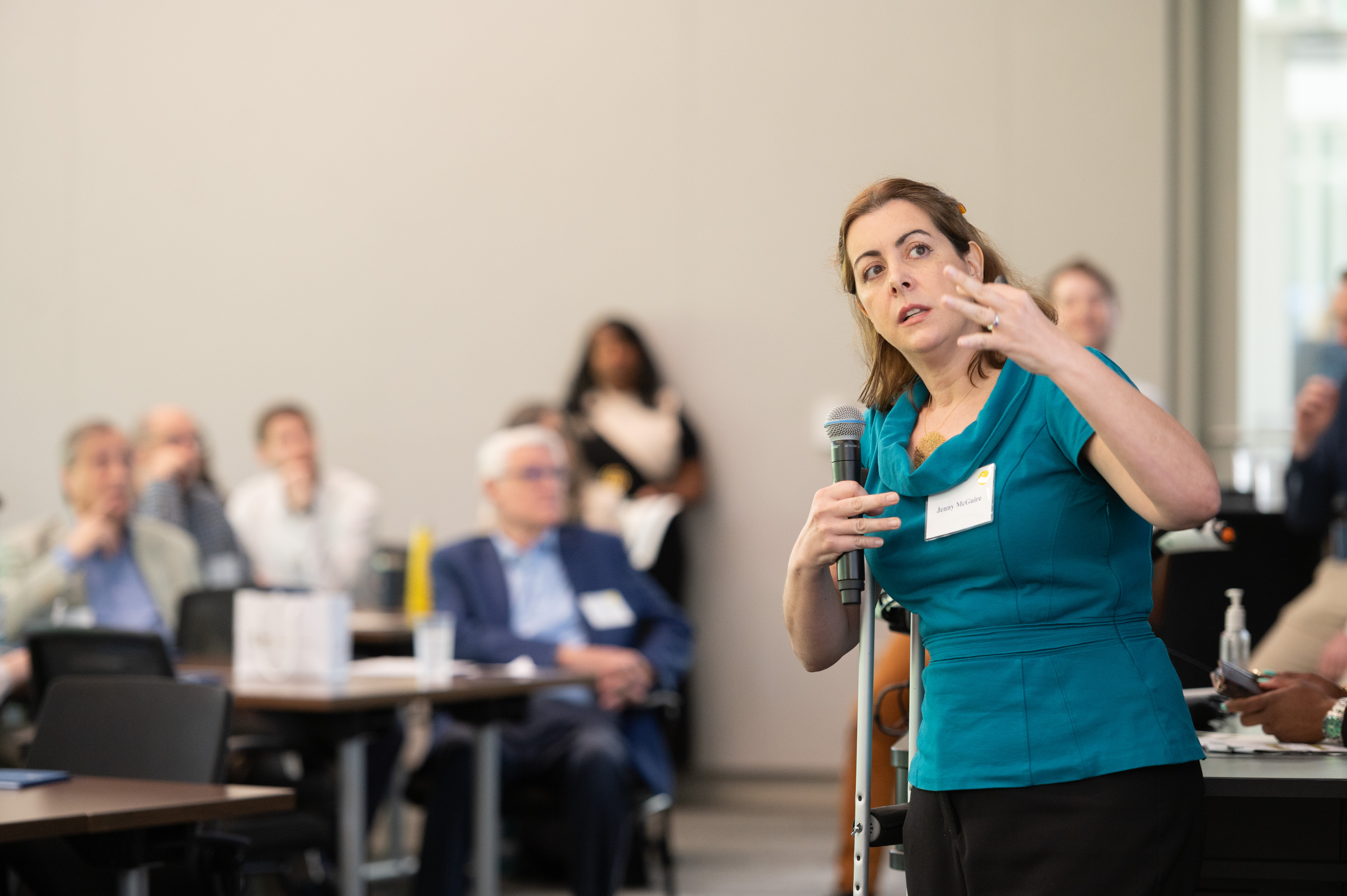
Jenny McGuire
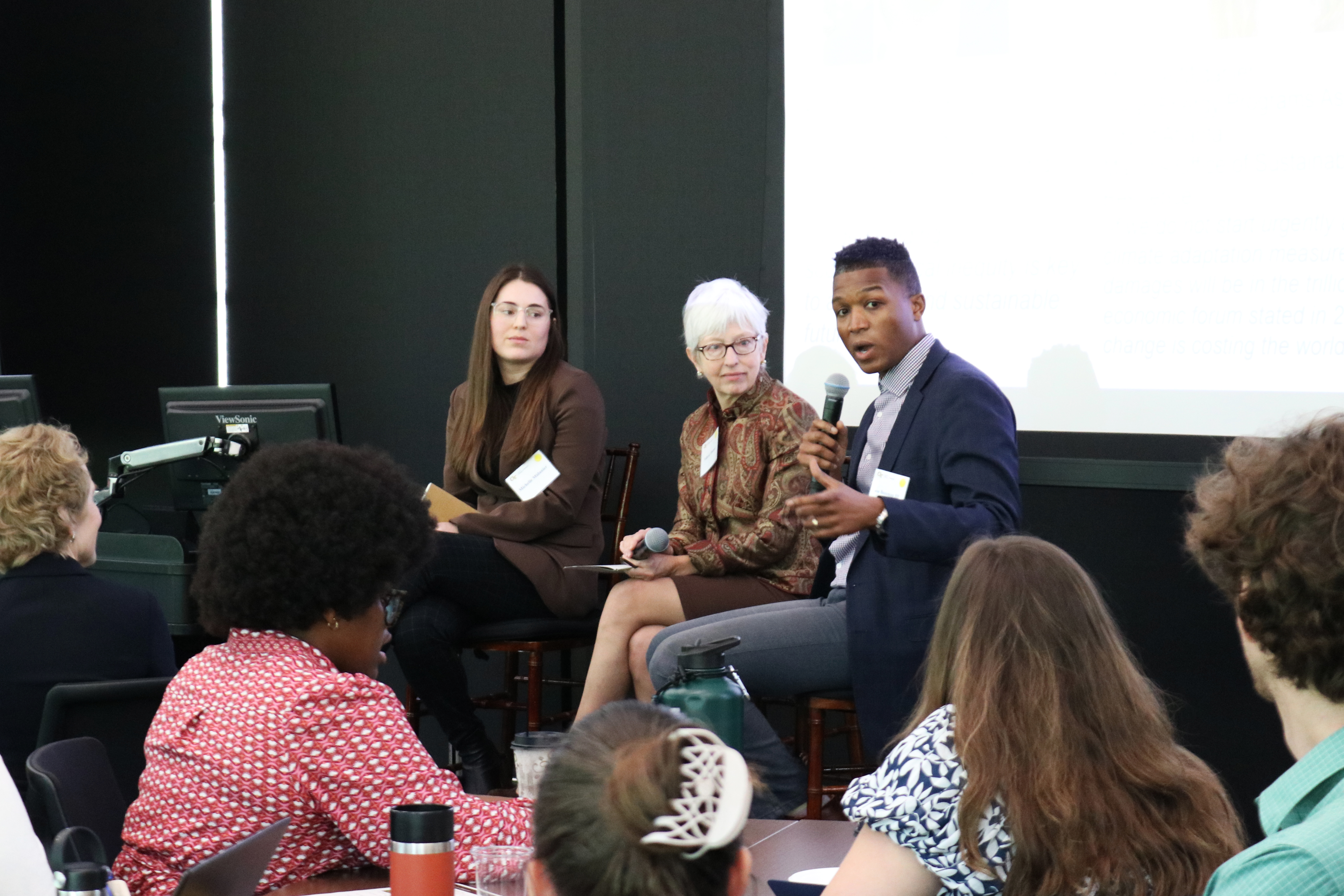
Frontiers in Science Policy Discussion Panelists: Michelle Midanier, Valerie Thomas and Joe F. Bozeman III
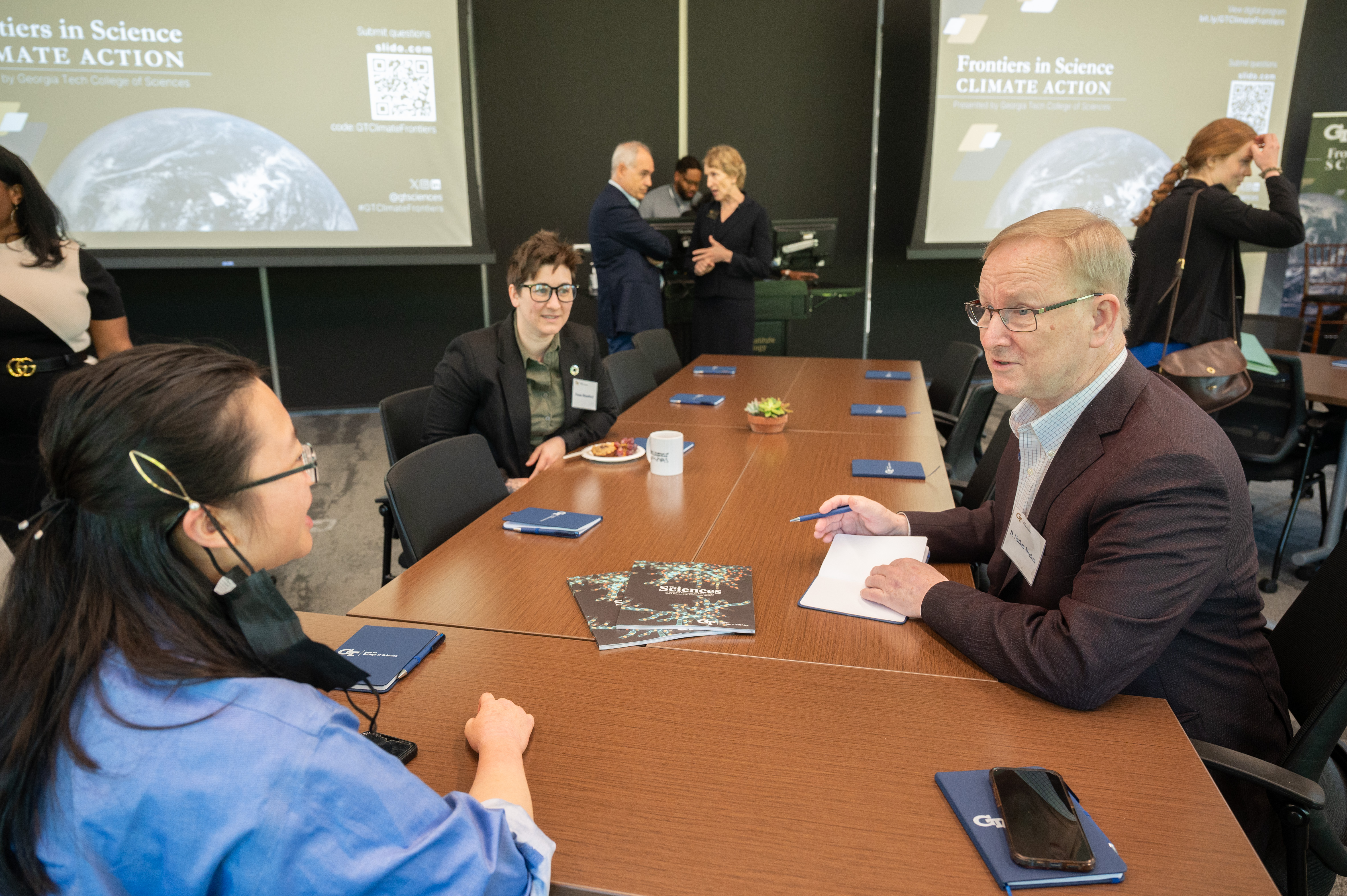
Frontiers in Science Participants
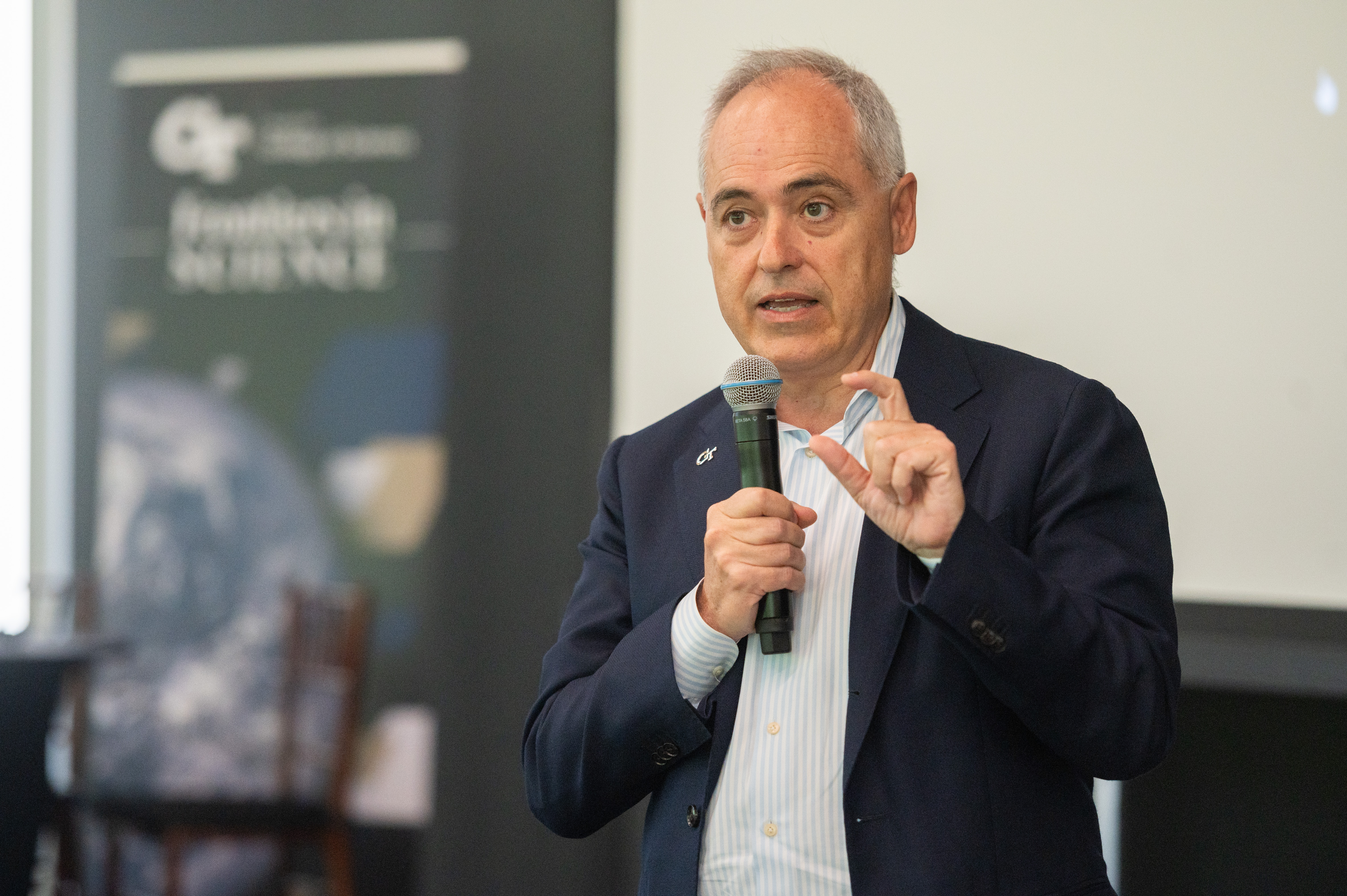
President Ángel Cabrera
By: Lindsay Vidal
Jess Hunt-Ralston
Director of Communications
College of Sciences at Georgia Tech
The Dynamics of Deformable Systems: Study Unravels Mathematical Mystery of Cable-like Structures
Feb 08, 2024 —
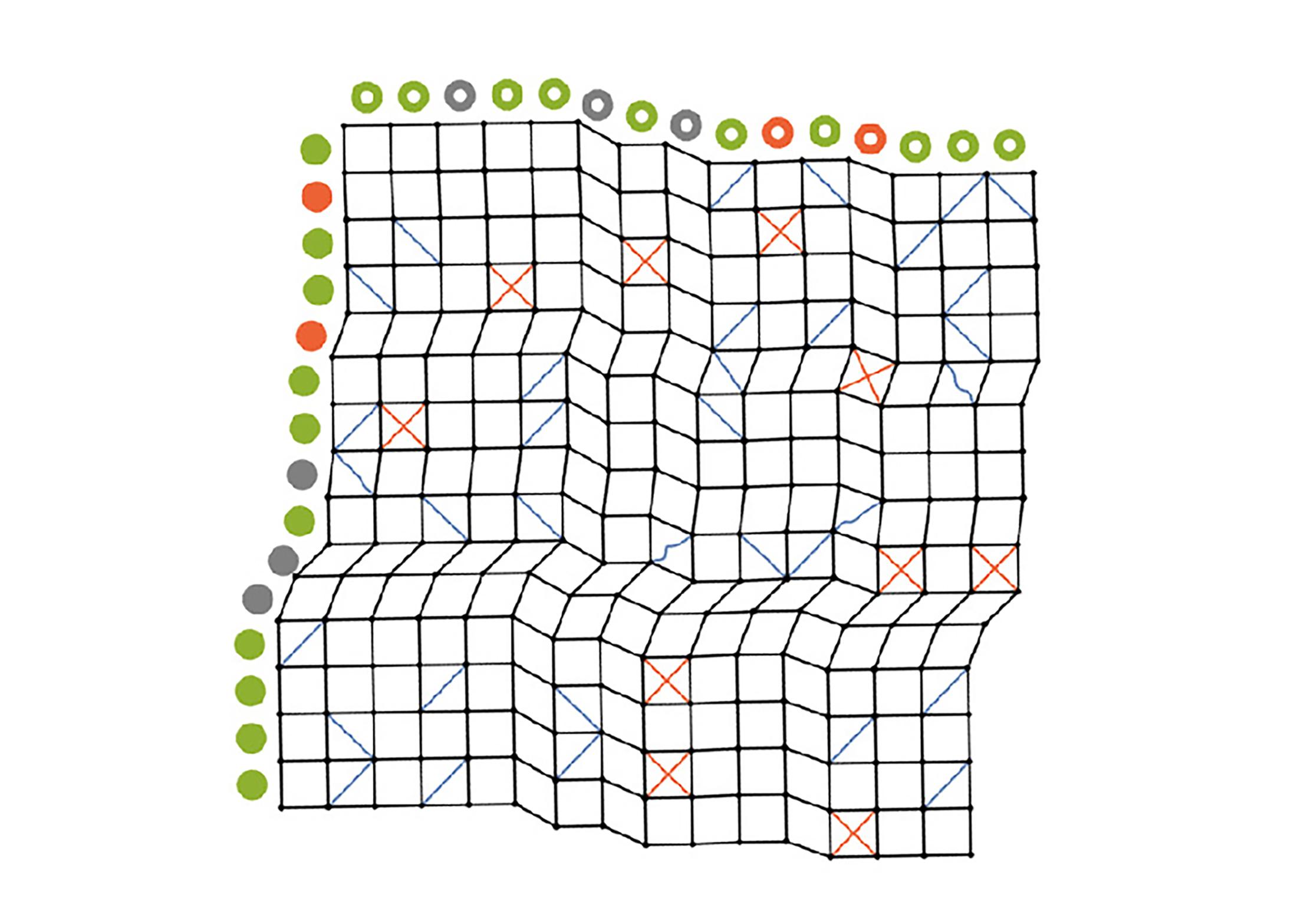
Systems of rigid rods acquire rigidity via the addition of random additional rods and cables, as captured via a graph theory. The research team's main object of study, shown here, is structures that consist of large numbers of pores — arranged in columns and rows with cables and rods added at random.
Are our bodies solid or liquid? We all know the convention — that solids maintain their shapes, while liquids fill the containers they’re in. But often in the real world, those lines are blurred. Imagine walking on a beach. Sometimes the sand gives way under feet, deforming like a liquid, but when enough sand grains pack together, they can support weight like a solid surface.
Modeling these kinds of systems is notoriously difficult — but Zeb Rocklin, an assistant professor in the School of Physics at Georgia Tech, has written a new paper doing just that.
Rocklin’s study, “Rigidity percolation in a random tensegrity via analytic graph theory,” is published in the journal Proceedings of the National Academy of Sciences (PNAS). The results have the potential to impact fields spanning biology to engineering and nanotechnology, showing that these types of deformable solids offer a rare combination of durability and flexibility.
"I'm very proud of our team, especially Will and Vishal, the two Georgia Tech undergraduates who co-led the study,” Rocklin says.
The lead author, William Stephenson, and co-author Vishal Sudhakar both completed their undergraduate studies at the Institute during the time of this research. Stephenson is now a first-year grad student at the University of Michigan, Ann Arbor, and Sudhakar has been admitted to Georgia Tech as a graduate student. Additionally, co-author Michael Czajkowski is a post-doctoral researcher in the School of Physics, and co-author James McInerney completed his graduate studies in the School of Physics under Rocklin. McInerney is now a postdoctoral researcher at the University of Michigan.
Connecting the dots… with cables
Imagine building molecules in chemistry class — large wooden spheres connected with sticks or rods. While many models use rods, including mathematical models, biological systems in real life are constructed of polymers, which function more like stretchy strings.
Likewise, when creating mathematical or biological models, researchers frequently treat all the elements as rods as opposed to treating some of them as cables, or strings. But, “there are tradeoffs between how mathematically tractable a model is and how physically plausible it is,” Rocklin says. “Physicists can have some beautiful mathematical theories, but they aren’t always realistic.” For example, a model using connective rods might not capture the dynamics that connective strings provide. “With a string you can stretch it, and it'll fight you, but when you compress it, it collapses.”
“But, in this study, we’ve extended the current theories,” he says, adding cable-like elements. “And that actually turns out to be incredibly difficult, because these theories use mathematical equations. In contrast, the distance between the two ends of a cable is represented by an inequality, which is not an equation at all. So how do you create a mathematical theory when you aren't starting from equations?” While a rod has a certain length in a mathematical equation, the ends of the string have to be represented as less than or equal to a certain length.
In this situation “all the usual analytic theories completely break,” Rocklin says. “It becomes very difficult for physicists or for mathematicians.”
“The trick was to notice that these physical systems were logically equivalent to something called a directed graph,” Rocklin adds, “where different modes of deformation are linked to each other in specific ways. This allows us to take a relatively complicated system and massively compress it to a much smaller system. And when we did that, we were able to turn it into something that becomes extremely easy for the computer to do.”
From biology to engineering
Rocklin’s team found that when modeling with cables and springs, the target range changed — becoming softer, with a wider margin for error. “That could be really important for something like a biological system, because a biological system is trying to stay close to that critical point,” says Rocklin. “Our model shows that the region around the critical point is actually much broader than what models that only used rods previously showed.”
Rocklin also points out applications for engineers. For example, since Rocklin's new theory suggests that even disordered cable structures can be strong and flexible, it may help engineers leverage cables as building materials to create safer, more durable bridges. The theory also provides a way to easily model these cable-based structures, to ensure their safety before they are built, and provide a way for engineers to iterate on designs.
Rocklin also notes potential applications in nanotechnology. “In nanotechnology, you must accept an increasing amount of disorder, because you can't just have a skilled worker actually go in and put segments there, and you can't have a conventional factory machine put segments there,” Rocklin says.
But biology has known how to lay down effective, but disordered, rod and cable structures for hundreds of millions of years. “This is going to tell us what sorts of machines we can make with those disordered structures when we're getting to the point of being able to do what biology can do. And that's a possible future design principle for the engineers to explore, at very small scales, where we can't choose exactly where each cable goes,” Rocklin says.
“Our theory shows that with cables, we can maintain a combination of flexibility and strength with much less precision than you might otherwise need.”
Funding: This research was funded by the Army Research Office through the MURI program (#W911NF2210219).
DOI: https://doi.org/10.1073/pnas.2302536120
Figure caption: Systems of rigid rods acquire rigidity via the addition of random additional rods and cables, as captured via a graph theory. The research team's main object of study, shown here, is structures that consist of large numbers of pores — arranged in columns and rows with cables and rods added at random.
Written by Selena Langner
Editor and Contact: Jess Hunt-Ralston
Researchers Leverage AI to Develop Early Diagnostic Test for Ovarian Cancer
Jan 29, 2024 —

Micrograph of a mucinous ovarian tumor (Photo National Institutes of Health)
For over three decades, a highly accurate early diagnostic test for ovarian cancer has eluded physicians. Now, scientists in the Georgia Tech Integrated Cancer Research Center (ICRC) have combined machine learning with information on blood metabolites to develop a new test able to detect ovarian cancer with 93 percent accuracy among samples from the team’s study group.
John McDonald, professor emeritus in the School of Biological Sciences, founding director of the ICRC, and the study’s corresponding author, explains that the new test’s accuracy is better in detecting ovarian cancer than existing tests for women clinically classified as normal, with a particular improvement in detecting early-stage ovarian disease in that cohort.
The team’s results and methodologies are detailed in a new paper, “A Personalized Probabilistic Approach to Ovarian Cancer Diagnostics,” published in the March 2024 online issue of the medical journal Gynecologic Oncology. Based on their computer models, the researchers have developed what they believe will be a more clinically useful approach to ovarian cancer diagnosis — whereby a patient’s individual metabolic profile can be used to assign a more accurate probability of the presence or absence of the disease.
“This personalized, probabilistic approach to cancer diagnostics is more clinically informative and accurate than traditional binary (yes/no) tests,” McDonald says. “It represents a promising new direction in the early detection of ovarian cancer, and perhaps other cancers as well.”
The study co-authors also include Dongjo Ban, a Bioinformatics Ph.D. student in McDonald’s lab; Research Scientists Stephen N. Housley, Lilya V. Matyunina, and L.DeEtte (Walker) McDonald; Regents’ Professor Jeffrey Skolnick, who also serves as Mary and Maisie Gibson Chair in the School of Biological Sciences and Georgia Research Alliance Eminent Scholar in Computational Systems Biology; and two collaborating physicians: University of North Carolina Professor Victoria L. Bae-Jump and Ovarian Cancer Institute of Atlanta Founder and Chief Executive Officer Benedict B. Benigno. Members of the research team are forming a startup to transfer and commercialize the technology, and plan to seek requisite trials and FDA approval for the test.
Silent killer
Ovarian cancer is often referred to as the silent killer because the disease is typically asymptomatic when it first arises — and is usually not detected until later stages of development, when it is difficult to treat.
McDonald explains that while the average five-year survival rate for late-stage ovarian cancer patients, even after treatment, is around 31 percent — but that if ovarian cancer is detected and treated early, the average five-year survival rate is more than 90 percent.
“Clearly, there is a tremendous need for an accurate early diagnostic test for this insidious disease,” McDonald says.
And although development of an early detection test for ovarian cancer has been vigorously pursued for more than three decades, the development of early, accurate diagnostic tests has proven elusive. Because cancer begins on the molecular level, McDonald explains, there are multiple possible pathways capable of leading to even the same cancer type.
“Because of this high-level molecular heterogeneity among patients, the identification of a single universal diagnostic biomarker of ovarian cancer has not been possible,” McDonald says. “For this reason, we opted to use a branch of artificial intelligence — machine learning — to develop an alternative probabilistic approach to the challenge of ovarian cancer diagnostics.”
Metabolic profiles
Georgia Tech co-author Dongjo Ban, whose thesis research contributed to the study, explains that “because end-point changes on the metabolic level are known to be reflective of underlying changes operating collectively on multiple molecular levels, we chose metabolic profiles as the backbone of our analysis.”
“The set of human metabolites is a collective measure of the health of cells,” adds coauthor Jeffrey Skolnick, “and by not arbitrary choosing any subset in advance, one lets the artificial intelligence figure out which are the key players for a given individual.”
Mass spectrometry can identify the presence of metabolites in the blood by detecting their mass and charge signatures. However, Ban says, the precise chemical makeup of a metabolite requires much more extensive characterization.
Ban explains that because the precise chemical composition of less than seven percent of the metabolites circulating in human blood have, thus far, been chemically characterized, it is currently impossible to accurately pinpoint the specific molecular processes contributing to an individual's metabolic profile.
However, the research team recognized that, even without knowing the precise chemical make-up of each individual metabolite, the mere presence of different metabolites in the blood of different individuals, as detected by mass spectrometry, can be incorporated as features in the building of accurate machine learning-based predictive models (similar to the use of individual facial features in the building of facial pattern recognition algorithms).
“Thousands of metabolites are known to be circulating in the human bloodstream, and they can be readily and accurately detected by mass spectrometry and combined with machine learning to establish an accurate ovarian cancer diagnostic,” Ban says.
A new probabilistic approach
The researchers developed their integrative approach by combining metabolomic profiles and machine learning-based classifiers to establish a diagnostic test with 93 percent accuracy when tested on 564 women from Georgia, North Carolina, Philadelphia and Western Canada. 431 of the study participants were active ovarian cancer patients, and while the remaining 133 women in the study did not have ovarian cancer.
Further studies have been initiated to study the possibility that the test is able to detect very early-stage disease in women displaying no clinical symptoms, McDonald says.
McDonald anticipates a clinical future where a person with a metabolic profile that falls within a score range that makes cancer highly unlikely would only require yearly monitoring. But someone with a metabolic score that lies in a range where a majority (say, 90%) have previously been diagnosed with ovarian cancer would likely be monitored more frequently — or perhaps immediately referred for advanced screening.
Citation: https://doi.org/10.1016/j.ygyno.2023.12.030
Funding
This research was funded by the Ovarian Cancer Institute (Atlanta), the Laura Crandall Brown Foundation, the Deborah Nash Endowment Fund, Northside Hospital (Atlanta), and the Mark Light Integrated Cancer Research Student Fellowship.
Disclosure
Study co-authors John McDonald, Stephen N. Housley, Jeffrey Skolnick, and Benedict B. Benigno are the co-founders of MyOncoDx, Inc., formed to support further research, technology transfer, and commercialization for the team’s new clinical tool for the diagnosis of ovarian cancer.
Writer: Renay San Miguel
Communications Officer II/Science Writer
College of Sciences
404-894-5209
Editor: Jess Hunt-Ralston
Smart Solids: Zeb Rocklin Awarded NSF CAREER for Flexible Metamaterials Research
Dec 12, 2023 —

Imagine materials that respond to their environment: winter jackets that become thicker as temperatures drop, shoes that return energy with each stride, and robots that adapt to better accomplish their task as they aid in space exploration. All of these ideas could be made into a reality through mechanical metamaterials, a group of flexible solids that blur the traditional definition of what a solid is.
Understanding these metamaterials is key to “programming” them correctly, maximizing their utility. “One of the paradigms of this research is that the material is the machine,” Zeb Rocklin, an assistant professor in the School of Physics, explains. “We're creating a material that performs the mechanical tasks that we want it to, and the processes, forces and displacements in the ways we want it to.”
A new $630,000 NSF CAREER grant will help Rocklin continue that research.
The National Science Foundation Faculty Early Career Development Award is a five-year grant designed to help promising researchers establish a foundation for a lifetime of leadership in their field. Known as CAREER awards, the grants are NSF’s most prestigious funding for untenured assistant professors.
The award, for “Geometric and topological mechanics of flexible structures,” will help Rocklin continue developing a new, unified theory for mechanical metamaterials — a group of structures that can flex and move, while having traditional solid components that make it easier to model. The theory could then be applied by other scientists and engineers to create responsive objects with smart fabrics that could respond to changes in environment — like novel knee replacements, responsive airplane wings, and better robots.
Materials as machines
“A solid is defined by the fact that it has a shape, and if I try to change the shape it might generate patterns of stress, or if I hit it, you might hear noise, because it's vibrating,” says Rocklin. “While we often think about things in terms of solids, liquids, and gasses, a lot of the things that are very important to us are not what we think of as a conventional solid.”
Flexible solids, like clothing, robots, and even our own bodies permeate our world, and are often some of the most useful materials we encounter. “This creates this huge challenge,” Rocklin says, “because flexible solids can't always be understood using current techniques of physics. We can write down the equations, but the equations are often too hard for anyone to solve.” For example, imagine trying to predict or replicate the infinite ways a piece of paper can crumple. As a result, flexible solids are often expensive and time consuming to model.
That’s where Rocklin’s new theory comes in.
Mechanical metamaterials
By combining well-known solids with flexible properties, Rocklin hopes to create a mathematically simple theory. “There are philosophical differences and limitations here,” he says, “but as a physicist, I’m looking for universal principles that can apply to a variety of things. Our technique is meant to complement the existing simulations, and it's meant to provide us more insight into these systems so that we can understand how to control them better.”
By building a theory around materials made of repeating solids connected by flexible hinges, Rocklin hopes to make a computationally inexpensive technique to predict and control the deformation of flexible structures. One example of this type of structure consists of solid square pieces connected by their corners in a checkerboard pattern. The pieces pivot against each other at these hinged corners, allowing the structure to easily expand and contract. “These materials find a sweet spot in between simple solids that were well-characterized in the nineteenth century and the flexible objects that are just too complicated for us to fully describe,” Rocklin adds.
While the material can only deform via one method, (by flexing at the hinges) this does not mean that there is only one way the material deforms. Rather, through this one method of deformation, there are an infinite number of modes or computations that the fabric can assume, illustrating Rocklin's key insight – that a single flexible mode inevitably gives rise to a whole host of complex deformations.
“There's very simple universal math to describe how this type of material operates,” Rocklin adds. “And, when people actually make this material, it turns out that it actually looks like this, and it actually deforms in this way.”
Broad applications
As a theoretical physicist, Rocklin is focused on developing a unified theory that can be applied by experts across many fields. For example, collapsable biomedical devices like stents, which should be small when inserted, but need to expand when inside the body. Inspired by the ever-adapting wings of birds, adaptable airplane wings are also an intriguing frontier.
Rather than minute adjustments via circuitry, airplane wings could be built from these flexible solids, which could be designed to automatically adapt when given a signal from the wind. Building an antenna from materials that respond to certain electromagnetic frequencies, to optimize signal reception, is another of many possible applications for the work.
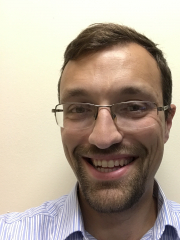
Written by Selena Langner
Contact: Jess Hunt- Ralston
American Mathematical Society Honors Trio of Faculty with Top Research Prize, Fellows Recognitions
Nov 22, 2023 —

Jennifer Hom
The American Mathematical Society (AMS) recently announced top honors for three School of Mathematics professors, including a top research award and a pair of faculty recognized as AMS Fellows for their work in advancing the field.
Levi L. Conant Prize
School of Mathematics Associate Professor Jennifer (Jen) Hom has received the 2024 Levi L. Conant Prize from the AMS. The award recognizes the best expository paper published in either Notices of the AMS or Bulletin of the AMS in the preceding five years. Hom is recognized for her paper, “Getting a handle on the Conway knot,” which was published in the Bulletin in 2021.
“These awards partially signal the depth and breadth of accomplishment and influence of these three remarkable scholars," says School of Mathematics Chair Mike Wolf. "Jen’s tribute is for her how she was able to communicate the clarity of her understanding of fundamental and difficult mathematics to a wide audience, creating a resource that will affect mathematics and mathematicians for many years."
“I am honored to receive the 2024 Levi L. Conant Prize,” Hom says. “An extremely important but often undervalued part of our job as mathematicians is communication, and I’m grateful to the AMS for valuing high-quality exposition in their publications.”
Hom adds that she’s “proud to be in the company of my esteemed former colleague Dan Margalit,” who won the Conant Prize in 2021. Margalit then served as a Mathematics Professor at Georgia Tech, and is now Stephenson Professor and Chair of the Department of Mathematics at Vanderbilt University.
AMS cited Hom’s article as “a wonderful resource for the community on timely and important material,” adding that “Hom’s paper packs a remarkable amount of knot theory into 11 pages, but remains clear, engaging, and easy to read throughout. Readers are left with new understanding and a sense of excitement for the future of this field.”
American Mathematical Society Fellows
Last year, Hom was also recognized as an AMS Fellow for her topology research and service to the mathematical community.
This season, two fellow School of Mathematics faculty, Professor Greg Blekherman and Professor Thang Le, also have joined those ranks.
“Thang and Greg received a distinction reserved for only the top few percent of research mathematicians nationwide," Wolf says. "Thang was singled out for his deep work in the creation and development of the fairly new subject of quantum topology over the last quarter century as well as for that subject’s implications for the very classic area of low-dimensional topology. Quantum topology is now a vast area, but many of its most prominent achievements came about through the work of Thang.
“Greg’s work courses through and blends algebraic and convex geometry as well as combinatorics and optimization — and also mathematical biology," Wolf explains. "Most notably, Greg is known for his diverse and important contributions to the theory of nonnegative and “sum of squares” polynomials, a hugely important topic in contemporary optimization theory.
Blekherman and Le are among more than three dozen mathematical scientists from around the world named 2024 AMS Fellows — a cohort which also includes Kasso Okoudjou, a former School of Mathematics Ph.D. student advised by Professor Christopher Heil.
“It is my pleasure to congratulate and welcome the new class of AMS Fellows, honored for their outstanding contributions to the mathematical sciences and to our profession,” notes AMS President Bryna Kra. "This year's class was selected from a large and excellent pool of candidates, highlighting the many ways in which our profession is advanced, and I look forward to working with them in service to our community."
“The school is just thrilled by these well-deserved awards to our wonderful colleagues," Wolf added.
About Jennifer (Jen) Hom
Hom joined Georgia Tech as an assistant professor in 2015 after she served as a Ritt Assistant Professor at Columbia University. She has been an associate professor in the School of Mathematics since 2018. Hom’s research centers on low-dimensional topology, which she usually studies using Heegaard Floer homology. She was asked to speak in the topology section of the 2022 International Congress of Mathematicians, the world’s largest gathering of mathematicians. Hom has held a Sloan Fellowship and a Simons Fellowship, is an AMS Fellow, and holds a National Science Foundation CAREER award.
About Greg Blekherman
Blekherman, who joined Georgia Tech in 2011, is a 2012 recipient of the Sloan Research Fellowship. His research interests lie at the intersection of convex and algebraic geometry. Blekherman received his Ph.D. in 2005 from the University of Michigan under the direction of Alexander Barvinok, and he has held postdoctoral positions at the Microsoft Research Theory Group, Virginia Bioinformatics Institute, Institute for Pure and Applied Math (UCLA) and UC San Diego before joining Georgia Tech.
About Thang Le
Le received his M.S. and Ph.D. from Lomonosov Moscow State University, and joined Georgia Tech in 2003. His research interests include differential topology, 3-manifolds, knot theory, and quasicrystals. He serves as an editor of Quantum Topology, The Journal of Knot Theory and Its Ramifications, and Acta Mathematica Vietnamica.
Read the AMS press releases on the 2024 Conant Prize and AMS Fellows here.
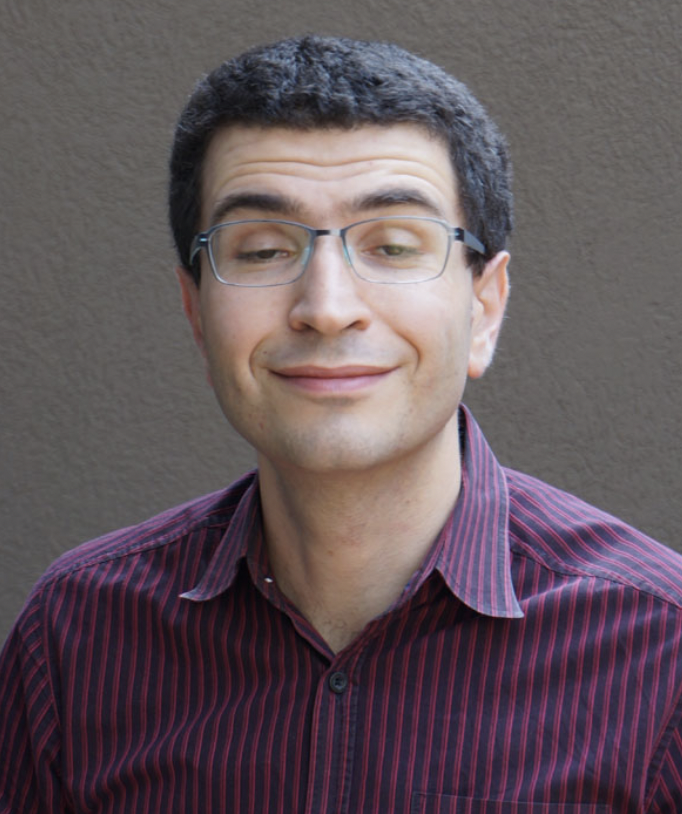
Greg Blekherman
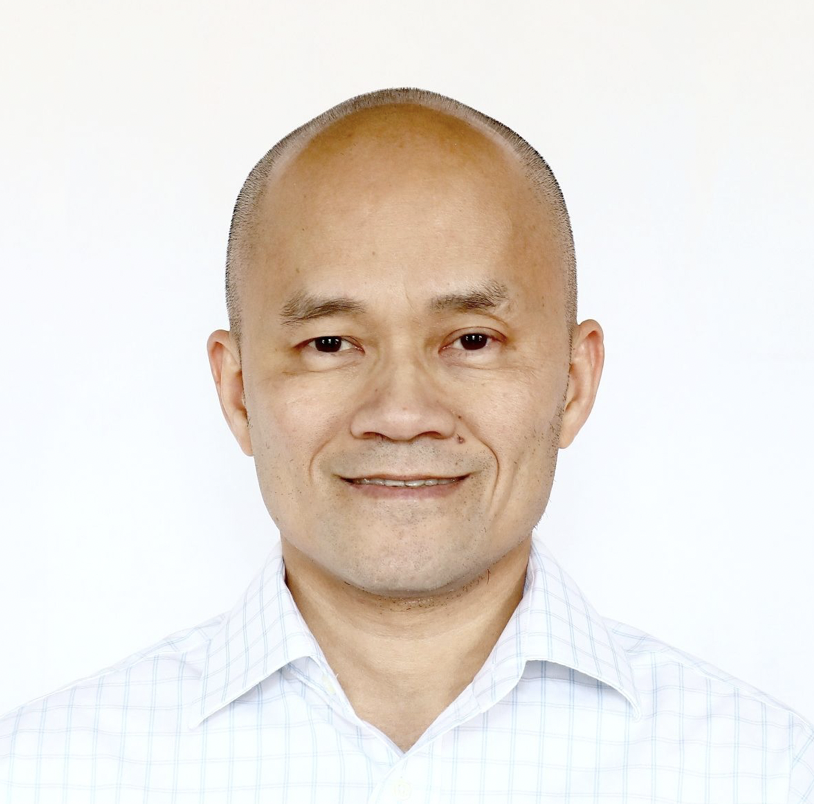
Thang Le
Writer: Renay San Miguel
Communications Officer II/Science Writer
College of Sciences
404-894-5209
Editor: Jess Hunt-Ralston
Study Reveals Wintertime Formation of Large Pollution Particles in China’s Skies
Nov 16, 2023 —

Beijing pollution (Photo Kevin Dooley, Creative Commons)
Previous studies have found that the particles that float in the haze over the skies of Beijing include sulfate, a major source of outdoor air pollution that damages lungs and aggravates existing asthmatic symptoms, according to the California Air Resources Board.
Sulfates usually are produced by atmospheric oxidation in the summer, when ample sunlight facilitates the oxidation that turns sulfur dioxide into dangerous aerosol particles. How is it that China can produce such extreme pollution loaded with sulfates in the winter, when there’s not as much sunlight and atmospheric oxidation is slow?
Yuhang Wang, professor in the School of Earth and Atmospheric Sciences at Georgia Tech, and his research team have conducted a study that may have the answer: All the chemical reactions needed to turn sulfur dioxide into sulfur trioxide, and then quickly into sulfate, primarily happen within the smoke plumes causing the pollution. That process not only creates sulfates in the winter in China, but it also happens faster and results in larger sulfate particles in the atmosphere.
“We call the source ‘in-source formation,’” Wang says. “Instead of having oxidants spread out in the atmosphere, slowly oxidizing sulfur dioxide into sulfur trioxide to produce sulfate, we have this concentrated production in the exhaust plumes that turns the sulfuric acid into large sulfate particles. And that's why we're seeing these large sulfate particles in China.”
The findings of in-source formation of larger wintertime sulfate particles in China could help scientists accurately assess the impacts of aerosols on radiative forcing — how climate change and global warming impact the Earth’s energy and heat balances — and on health, where larger aerosols means larger deposits into human lungs.
“Wintertime Formation of Large Sulfate Particles in China and Implications for Human Health,” is published in Environmental Science & Technology, an American Chemical Society publication. The co-authors include Qianru Zhang of Peking University and Mingming Zheng of Wuhan Polytechnic University, two of Wang’s former students who conducted the research while at Georgia Tech.
Explaining a historic smog
China still burns a lot of coal in power plants because its costs are lower compared to natural gas, Wang says. It also makes for an easy comparison between China’s hazy winters and a historic event that focused the United Kingdom’s attention on dangerous environmental hazards — the Great London Smog.
The event, depicted in the Netflix show “The Crown,” saw severe smog descend on London in December 1952. Unusually cold weather preceded the event, which brought the coal-produced haze down to ground level. UK officials later said the Great London Smog (also called the Great London Fog) was responsible for 4,000 deaths and 100,000 illnesses, although later studies estimated a higher death toll of 10,000 to 20,000.
“From the days of the London Fog to extreme winter pollution in China, it has been a challenge to explain how sulfate is produced in the winter,” Wang says.
Wang and his team decided to take on that challenge.
Aerosol size and heavy metal influence?
The higher sulfate levels in China, notably in January 2013, defy conventional explanations that relied on standard photochemical oxidation. It was thought that nitrogen dioxide or other mild oxidants found in alkaline or neutral particles in the atmosphere were the cause. But measurements revealed the resulting sulfate particles were highly acidic.
During Zheng’s time at Georgia Tech, “She was just looking for interesting things to do,” Wang says of the former student. “And I said, maybe this is what we should do — I wanted her to look at aerosol size distributions, how large the aerosols are.”
Zheng and Wang noticed that the size of the sulfate particles from China’s winter were much larger than those that resulted from photochemically-produced aerosols. Usually measuring 0.3 to 0.5 microns, the sulfate was closer to 1 micron in size. (A human hair is about 70 microns.) Aerosols distributed over a wider area would normally be smaller.
“The micron-sized aerosol observations imply that sulfate particles undergo substantial growth in a sulfur trioxide-rich environment,” Wang says. Larger particles increase the risks to human health.
“When aerosols are large, more is deposited in the front part of the respiratory system but less on the end part, such as alveoli,” he adds. “When accounting for the large size of particles, total aerosol deposition in the human respiratory system is estimated to increase by 10 to 30 percent.”
Something still needs to join the chemical mix, however, so the sulfur dioxide could turn into sulfur trioxide while enlarging the resulting sulfate particles. Wang says a potential pathway involves the catalytic oxidation of sulfur dioxide to sulfuric acid by “transition metals.”
High temperatures, acidity, and water content in the exhaust can greatly accelerate catalytic sulfur dioxide oxidation “compared to that in the ambient atmosphere. It is possible that similar heterogeneous processes occurring on the hot surface of a smokestack coated with transition metals could explain the significant portion of sulfur trioxide observed in coal-fired power plant exhaust,” Wang says.
“A significant amount of sulfur trioxide is produced, either during combustion or through metal-catalyzed oxidation at elevated temperatures.”
An opportunity for cleaner-burning coal power plants
The impact of in-source formation of sulfate suggests that taking measures to cool off and remove sulfur trioxide, sulfuric acid, and particulates from the emissions of coal-combustion facilities could be a way to cut down on pollution that can cause serious health problems.
“The development and implementation of such technology will benefit nations globally, particularly those heavily reliant on coal as a primary energy source,” Wang says.
DOI: https://doi.org/10.1021/acs.est.3c05645
Funding: This study was funded by the National Natural Science Foundation of China (nos. 41821005 and 41977311). Yuhang Wang was supported by the National Science Foundation Atmospheric Chemistry Program. Qianru Zhang would also like to thank the China Postdoctoral Science Foundation (2022M720005) and China Scholarship Council for support. Mingming Zheng is also supported by the Fundamental Research Funds for the Central Universities, Peking University (7100604309).
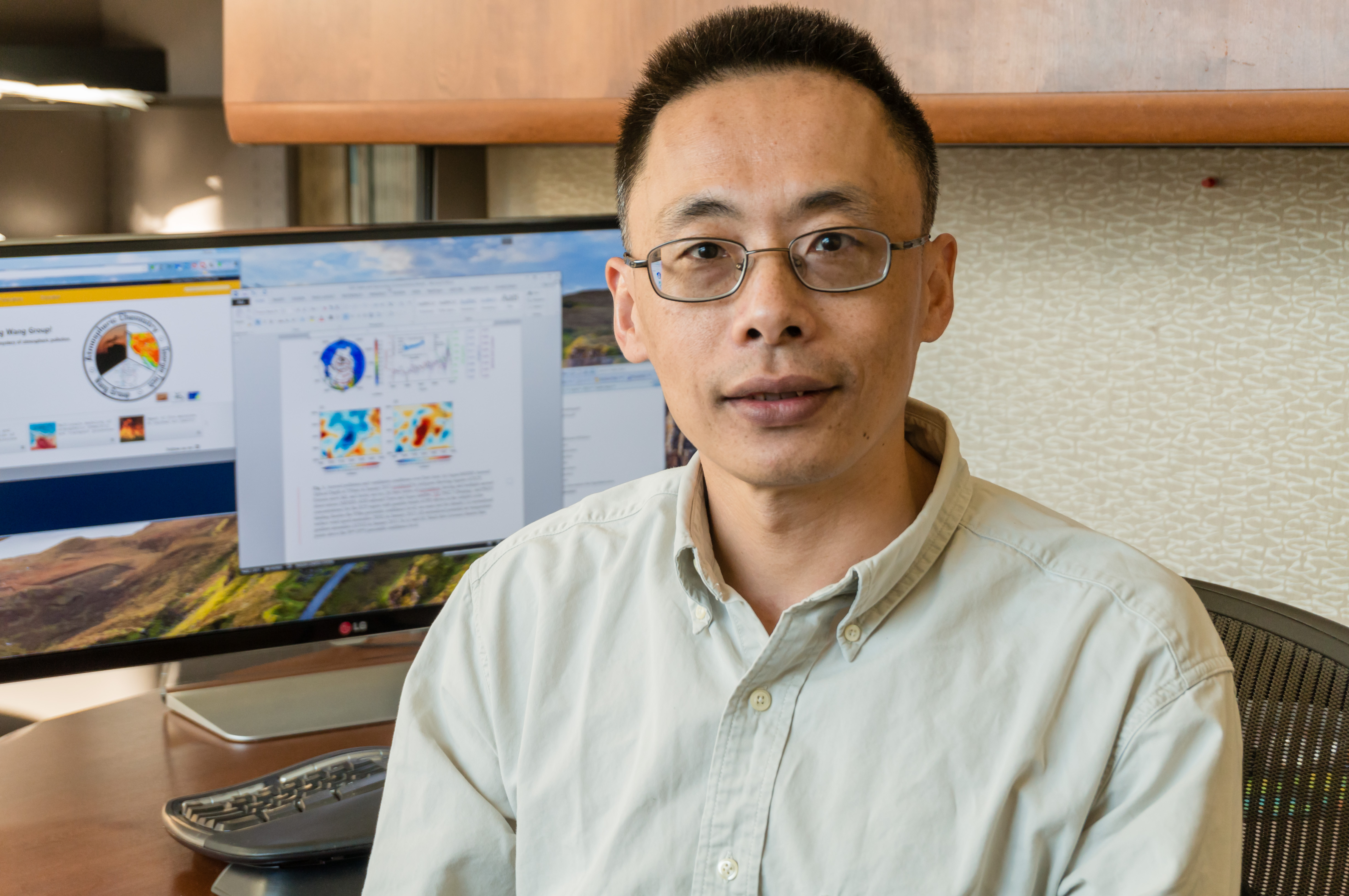
Yuhang Wang
Writer: Renay San Miguel
Communications Officer II/Science Writer
College of Sciences
404-894-5209
Editor: Jess Hunt-Ralston