26th NanoFANS Forum
26th NanoFANS Forum
Micro- and Nanotechnology Commercialization: Opportunities and Challenges - Part 2
AGENDA:
The Global Center for Medical Innovation Virtual Brown-bag Q&A
The Office of the VPIR presents:
The Global Center for Medical Innovation
Virtual Brown-bag Q&A
Please join us learn more about the work of this vital Georgia Tech resource for biomedical research.
New Wearable Device Measures Tumors Changing Size Below Skin
Sep 16, 2022 — Atlanta, GA

Engineers at the Georgia Institute of Technology and Stanford University have created a small, autonomous device with a stretchable/flexible sensor that can be adhered to the skin to measure the changing size of tumors below. The non-invasive, battery-operated device is sensitive to one-hundredth of a millimeter (10 micrometers) and can beam results to a smartphone app wirelessly in real-time with the press of a button.
In practical terms, the researchers say, their device—dubbed FAST for “Flexible Autonomous Sensor measuring Tumors”—represents a wholly new, fast, inexpensive, hands-free, and accurate way to test the efficacy of cancer drugs. On a grander scale, it could lead to promising new directions in cancer treatment.
Each year researchers test thousands of potential cancer drugs on mice with subcutaneous tumors. Few make it to human patients, and the process for finding new therapies is slow because technologies for measuring tumor regression from drug treatment take weeks to read out a response. The inherent biological variation of tumors, the shortcomings of existing measuring approaches, and the relatively small sample sizes make drug screenings difficult and labor-intensive.
“In some cases, the tumors under observation must be measured by hand with calipers,” says Alex Abramson, first author of the study and a recent post-doc in the lab of Zhenan Bao at the Stanford School of Engineering and now an assistant professor at Georgia Tech. The use of metal pincer-like calipers to measure soft tissues is not ideal, and radiological approaches cannot deliver the sort of continuous data needed for real-time assessment. FAST can detect changes in tumor volume on the minute-timescale, while caliper and bioluminescence measurements often require weeks-long observation periods to read out changes in tumor size.
FAST’s sensor is composed of a flexible and stretchable skin-like polymer that includes an embedded layer of gold circuitry. This sensor is connected to a small electronic backpack designed by former post-docs and co-authors Yasser Khan and Naoji Matsuhisa. The device measures the strain on the membrane—how much it stretches or shrinks—and transmits that data to a smartphone. Using the FAST backpack, potential therapies that are linked to tumor size regression can quickly and confidently be excluded as ineffective or fast-tracked for further study.
The researchers say that the new device offers at least three significant advances. First, it provides continuous monitoring, as the sensor is physically connected to the mouse and remains in place over the entire experimental period. Second, the flexible sensor enshrouds the tumor and is therefore able to measure shape changes that are difficult to discern with other methods. Third, FAST is both autonomous and non-invasive. It is connected to the skin, not unlike a band-aid, battery operated and connected wirelessly. The mouse is free to move unencumbered by the device or wires, and scientists do not need to actively handle the mice following sensor placement. FAST packs are also reusable, cost just $60 or so to assemble and can be attached to the mouse in minutes.
The breakthrough is in FAST’s flexible electronic material. Coated on top of the skin-like polymer is a layer of gold, which, when stretched, develops small cracks that change the electrical conductivity of the material. Stretch the material and number of cracks increases, causing the electronic resistance in the sensor to increase as well. When the material contracts, the cracks come back into contact and conductivity improves.
Both Abramson and co-author Naoji Matsuhisa, an associate professor at the University of Tokyo, characterized how these crack propagation and exponential changes in conductivity can be mathematically equated with changes in dimension and volume.
One hurdle the researchers had to overcome was the concern that the sensor itself might compromise measurements by applying undue pressure to the tumor, effectively squeezing it. To circumvent that risk, they carefully matched the mechanical properties of the flexible material to skin itself to make the sensor as pliant and as supple as real skin.
“It is a deceptively simple design,” Abramson says, “But these inherent advantages should be very interesting to the pharmaceutical and oncological communities. FAST could significantly expedite, automate and lower the cost of the process of screening cancer therapies.”
Story by Andrew Myers
Citation: Abramson et al., Sci. Adv. 8, eabn6550 (2022) DOI: 10.1126/sciadv.abn6550
Alex Abramson is now Assistant Professor of Chemical and Biomolecular Engineering at The Georgia Institute of Technology; Yasser Khan is Assistant Professor at the Ming Hsieh Department of Electrical and Computer Engineering at the University of Southern California; Carmel T. Chan is a former Senior Scientific Manager at Stanford University; Alana Mermin-Bunnell is a student at Stanford University; Naoji Matsuhisa is Associate Professor in the Institute of Industrial Science Department of Informatics and Electronics at the University of Tokyo; Robyn Fong is a Life Science Research Professor in the Cardiothoracic Surgery Department at Stanford University; Rohan Shad is a former Postdoctoral Fellow at Stanford University School of Medicine; William Hiesinger is Assistant Professor of Cardiothoracic Surgery at Stanford University; Parag Mallick is Associate Professor of Radiology at Stanford University; Zhenan Bao is the K.K. Lee Professor in Chemical Engineering at Stanford University.
The research was supported in part by an NIH F32 fellowship (Grant 1F32EB029787) and the Stanford Wearable Electronics Initiative (eWEAR).
The eWEAR-TCCI awards for science writing is a project commissioned by the Wearable Electronics Initiative (eWEAR) at Stanford University and made possible by funding through eWEAR industrial affiliates program member Shanda Group and the Tianqiao and Chrissy Chen Institute (TCCI®).
Tess Malone, Research Writer/Editor
Researchers Develop Painless Tattoos That Can Be Self-Administered
Sep 14, 2022 — Atlanta, GA
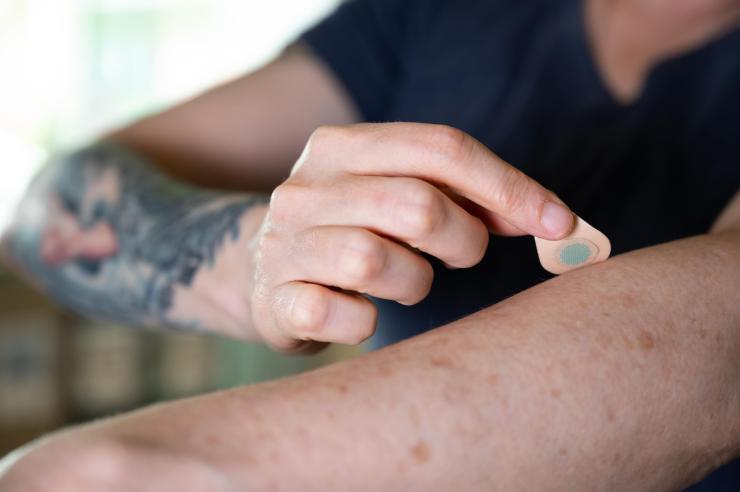
A microneedle patch tattoo is pressed to the skin.
Instead of sitting in a tattoo chair for hours enduring painful punctures, imagine getting tattooed by a skin patch containing microscopic needles. Researchers at the Georgia Institute of Technology have developed low-cost, painless, and bloodless tattoos that can be self-administered and have many applications, from medical alerts to tracking neutered animals to cosmetics.
“We’ve miniaturized the needle so that it’s painless, but still effectively deposits tattoo ink in the skin,” said Mark Prausnitz, principal investigator on the paper. “This could be a way not only to make medical tattoos more accessible, but also to create new opportunities for cosmetic tattoos because of the ease of administration.”
Prausnitz, Regents’ Professor and J. Erskine Love Jr. Chair in the School of Chemical and Biomolecular Engineering, presented the research in the journal iScience, with former Georgia Tech postdoctoral fellow Song Li as co-author.
Tattoos are used in medicine to cover up scars, guide repeated cancer radiation treatments, or restore nipples after breast surgery. Tattoos also can be used instead of bracelets as medical alerts to communicate serious medical conditions such as diabetes, epilepsy, or allergies.
Various cosmetic products using microneedles are already on the market — mostly for anti-aging — but developing microneedle technology for tattoos is new. Prausnitz, a veteran in this area, has studied microneedle patches for years to painlessly administer drugs and vaccines to the skin without the need for hypodermic needles.
“We saw this as an opportunity to leverage our work on microneedle technology to make tattoos more accessible,” Prausnitz said. “While some people are willing to accept the pain and time required for a tattoo, we thought others might prefer a tattoo that is simply pressed onto the skin and does not hurt.”
Transforming Tattooing
Tattoos typically use large needles to puncture repeatedly into the skin to get a good image, a time-consuming and painful process. The Georgia Tech team has developed microneedles that are smaller than a grain of sand and are made of tattoo ink encased in a dissolvable matrix.
“Because the microneedles are made of tattoo ink, they deposit the ink in the skin very efficiently,” said Li, the lead author of the study.
In this way, the microneedles can be pressed into the skin just once and then dissolve, leaving the ink in the skin after a few minutes without bleeding.
Tattooing Technique
Although most microneedle patches for pharmaceuticals or cosmetics have dozens or hundreds of microneedles arranged in a square or circle, microneedle patch tattoos imprint a design that can include letters, numbers, symbols, and images. By arranging the microneedles in a specific pattern, each microneedle acts like a pixel to create a tattoo image in any shape or pattern.
The researchers start with a mold containing microneedles in a pattern that forms an image. They fill the microneedles in the mold with tattoo ink and add a patch backing for convenient handling. The resulting patch is then applied to the skin for a few minutes, during which time the microneedles dissolve and release the tattoo ink. Tattoo inks of various colors can be incorporated into the microneedles, including black-light ink that can only be seen when illuminated with ultraviolet light.
Prausnitz’s lab has been researching microneedles for vaccine delivery for years and realized they could be equally applicable to tattoos. With support from the Alliance for Contraception in Cats and Dogs, Prausnitz’s team started working on tattoos to identify spayed and neutered pets, but then realized the technology could be effective for people, too.
The tattoos were also designed with privacy in mind. The researchers even created patches sensitive to environmental factors such as light or temperature changes, where the tattoo will only appear with ultraviolet light or higher temperatures. This provides patients with privacy, revealing the tattoo only when desired.
The study showed that the tattoos could last for at least a year and are likely to be permanent, which also makes them viable cosmetic options for people who want an aesthetic tattoo without risk of infection or the pain associated with traditional tattoos. Microneedle tattoos could alternatively be loaded with temporary tattoo ink to address short-term needs in medicine and cosmetics.
Microneedle patch tattoos can also be used to encode information in the skin of animals. Rather than clipping the ear or applying an ear tag to animals to indicate sterilization status, a painless and discreet tattoo can be applied instead.
“The goal isn’t to replace all tattoos, which are often works of beauty created by tattoo artists,” Prausnitz said. “Our goal is to create new opportunities for patients, pets, and people who want a painless tattoo that can be easily administered.”
Li, Song, Kim, Youngeun, Lee, Jeong Woo, Prausnitz, Mark R. Mircroneedle patch tattoos. iScience (2022).
Prausnitz has co-founded a company called Micron Biomedical that is developing microneedle patch technology, bringing it further into clinical trials, commercializing it, and ultimately making it available to patients.
Prausnitz and several other Georgia Tech researchers are inventors of the microneedle patch technology used in this study and have ownership interest in Micron Biomedical. They are entitled to royalties derived from Micron Biomedical’s future sales of products related to the research. These potential conflicts of interest have been disclosed and are overseen by Georgia Institute of Technology.

Medical alert tattoo: microneedle patch (above) and tattoo on skin (below).
Credit: Song Li, Georgia Tech
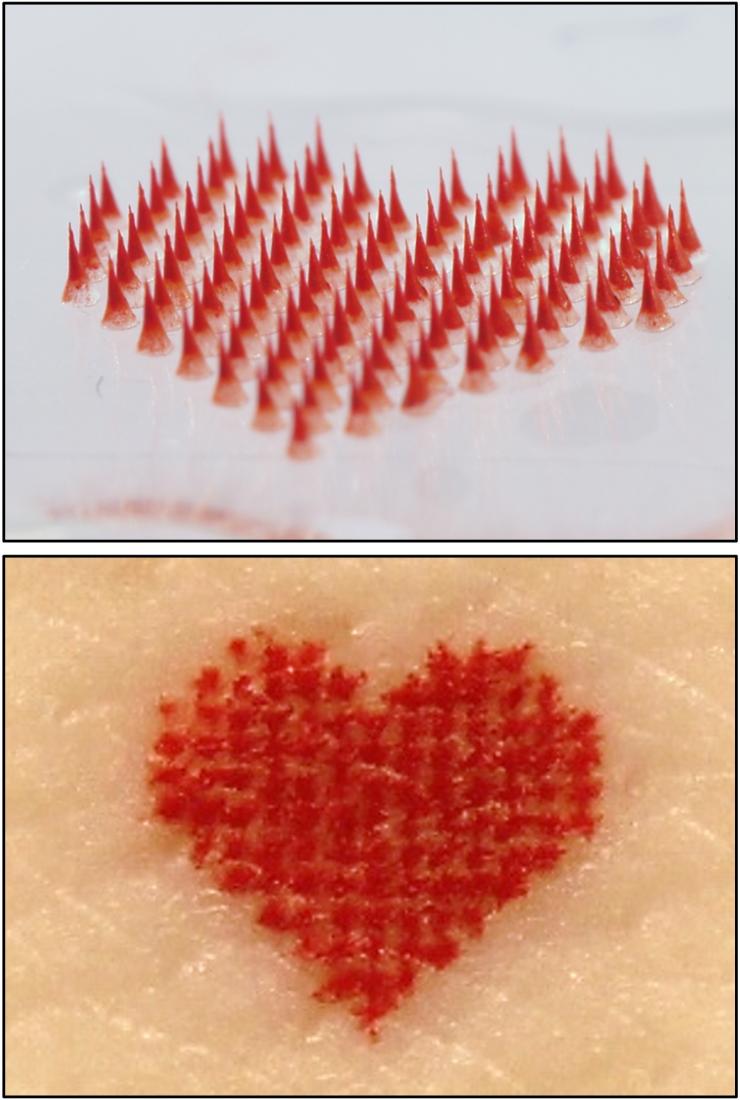
Heart tattoo: microneedle patch (above) and tattoo on skin (below).
Credit: Song Li, Georgia Tech
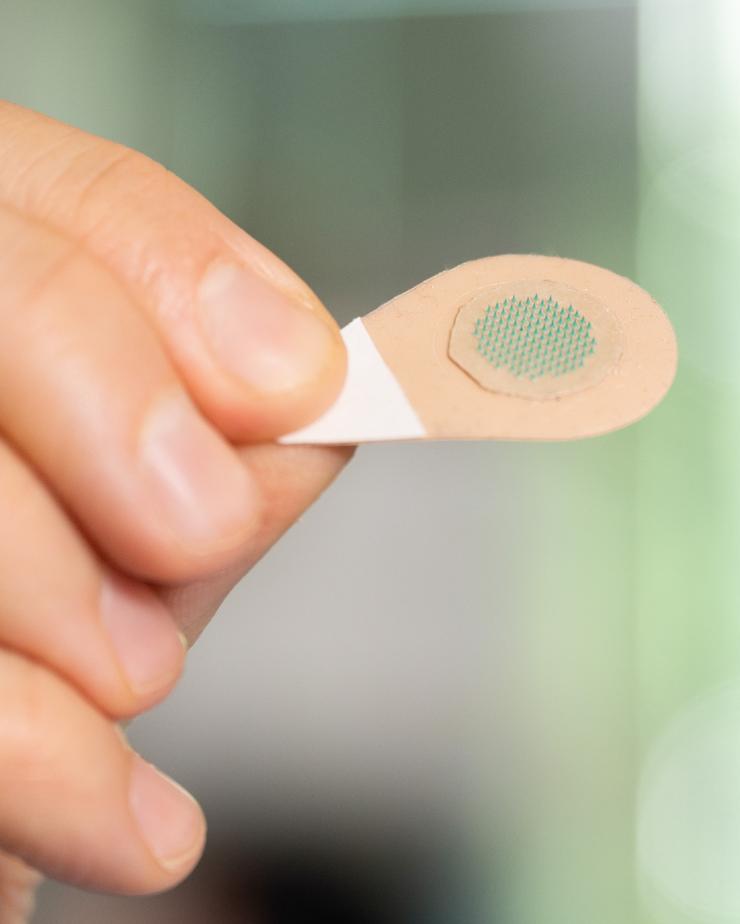
A magnified view of a microneedle patch with green tattoo ink.
Tess Malone, Research Writer/Editor
Cleanroom User Spotlight: Muneeb Zia
Sep 12, 2022 — Atlanta, GA
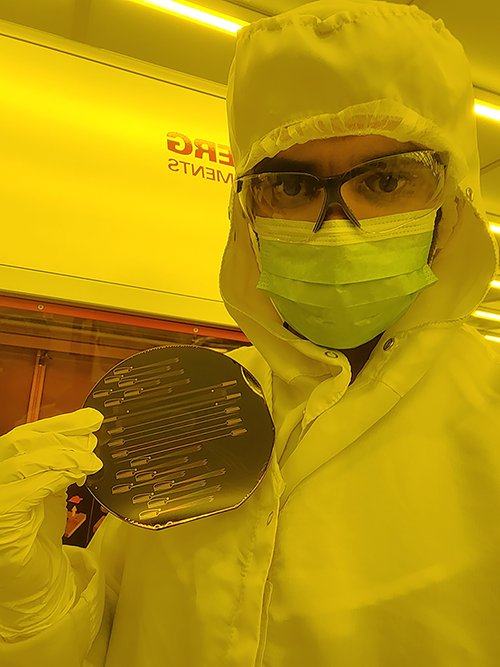
Muneeb Zia is a research engineer in the Integrated 3D Systems Lab led by Professor Muhannad Bakir. His research focuses on developing microfabrication technologies for heterogeneous integration and in vivo biosensing applications. In the following Q&A, Zia briefly discusses his work in the IEN cleanroom and gives advice to current and future users.
How long have you been using the IEN Cleanroom?
I have been using the IEN Cleanroom for about 10 years.
What tools do you use when you are in the cleanroom and what are you doing?
Most of my current work involves the fabrication of high-density flexible electromyography (EMG) electrodes. To do this, I use a variety of tools including, but not limited to:
- Spin coaters
- Heidelberg MLA 150 Maskless Aligner
- Mask Aligner
- Vision RIE
- STS PECVD
- CHA Evaporator
- Unifilm Sputterer
- Plasma Therm ICP
- Dektak 150 Profilometer
- Hitachi S-4700 FE-SEM
- ADT 7100 Dicing Saw
So, if you run into me in the cleanroom, I am probably making some flexible EMG electrodes.
What is/has been your favorite project you have worked on in the IEN cleanroom?
My favorite project has been the fabrication of flexible EMG electrodes. These flexible high-density arrays were initially developed to record single motor unit activity from the tiny muscles controlling breathing and vocal behavior in songbirds. However, since these initial studies, we have further developed our devices’ capabilities and collaborated with a number of labs to create new electrode designs. These new designs can record from several different species and muscle groups, including from humans. The first successful human trial was also carried out by the Pruszynski Lab in Canada earlier this year.
We are now working to share this technology with the greater neuroscience community around the world. We are conducting remote workshops where we provide the electrode arrays developed in the IEN Cleanroom along with online instruction materials to users to collect high-resolution EMG data in their own labs. To date, labs from around the world including labs in Canada, the United Kingdom, Japan, China, Switzerland, Portugal, Denmark, and Finland have participated in the workshop. We plan to have more than 100 labs around the world use the electrodes by the end of the workshop.
What would you say to other people thinking about using a tool in the IEN cleanroom?
Getting trained on a new tool is very streamlined and simple, and there are a lot of training videos available to get you started. The trainers in the IEN Cleanroom are also very accessible and always willing to work with you. So, do not wait!
What is your favorite thing about the IEN Cleanroom?
My favorite thing about the IEN Cleanroom is the extremely supportive and helpful staff.
XBAW Technology: Enabling Next Generation Resonators and Filter Solutions – from MHz to GHz range applications
Georgia Electronic Design Center Distinguished Lecture Series
XBAW Technology: Enabling Next Generation Resonators and Filter Solutions – from MHz to GHz range applications
Featuring Abhay Kochhar, Staff Device Engineer Akoustis Technologies, Inc.
2nd Materials for Energy Harvesting and Conversion Workshop
Center for Organic, Hybrid Photonics, and Electronics (COPE)
2nd Materials for Energy Harvesting and Conversion Workshop
Organic Electronics
11:30 – noon Jonathan Rivnay, Professor, Northwestern University
Noon – 12:30 Alexandra Paterson, Assistant Professor, University of Kentucky
12:30 – 13:00 Oana Jurchescu, Professor, Wake Forest University
NNCI Webinar: Innovation and Entrepreneurship
Lab-to-Fab: Transitioning from University Cleanrooms to Industrial Prototyping and Low-Volume Production
Featuring Miguel Urteaga, Director of Foundry Products and Services, Teledyne Scientific Company
Abstract: Emerging semiconductor technologies face many challenges in transitioning from initial technology demonstrations to successful products. This talk will discuss an important initial step of transitioning technologies from a university cleanroom into industrial prototyping and low-volume production necessary for initial product sampling.
NNCI Computation Webinar
Theoretical Exploration of Energy Efficient Spintronics Devices
Featuring Professor Tony Low | Department of Electrical and Computer Engineering, University of Minnesota
Abstract: With great advances by the spintronics device and materials communities, magnetic tunnel junctions (MTJ) have been demonstrated to be the only passive device that can function simultaneously for digital, memory, and analog in-memory computing, among other applications.
$65M Grant to Build AI Manufacturing in Georgia
Sep 06, 2022 — Atlanta, GA
Aaron Stebner (left) and Tom Kurfess are among the leaders of GA-AIM, a $65 million grant that focuses on manufacturing and AI in the state of Georgia.
Aaron Stebner outlined an aggressive plan for artificial intelligence and manufacturing when he applied for a faculty position in 2019. In his cover letter, he promised “to establish the Georgia Institute of Technology as a world leader in additive manufacturing of solid materials (ceramics & metals) R&D, especially in the fusion of data sciences and AI to create new, world-leading technologies.”
Stebner thought it would take 10-15 years of incremental steps and funding to achieve the goal. He was wrong.
Thanks to a new $65 million grant from the U.S. Department of Commerce’s Economic Development Administration, announced by President Joe Biden, Stebner’s plan will begin to become a reality — and include the entire state of Georgia and all of its manufacturing sectors from agriculture to airplanes — two years after arriving on campus.
The largest of the nine projects within the larger Georgia AI Manufacturing (GA-AIM) technology corridor grant will allow Stebner and Georgia Tech to transform the Advanced Manufacturing Pilot Facility (AMPF) into the Artificial Intelligence Manufacturing Pilot Facility (AI-MPF). The 24,000 square-foot facility on 14th Street will more than double in size after Georgia Tech and statewide GA-AIM partners were selected as one of 21 Phase II awardees in the $1 billion Build Back Better Regional Challenge (BBB) competition, part of the Investing in America’s Communities initiative under the American Rescue Plan Act of 2021.
AMPF has been a shell waiting for a vision like Build Back Better to fill it out,” said Stebner, associate professor the George W. Woodruff School of Mechanical Engineering and the School of Materials Science and Engineering. “Now we will transform the facility into one of the nation’s first manufacturing labs designed for autonomy with the goal of helping the state and the nation to be world AI manufacturing leaders.”
Read the entire story on the College of Engineering website.
Jason Maderer
College of Engineering
maderer@gatech.edu